Sandbox 30
From Proteopedia
Please do NOT make changes to this Sandbox. Sandboxes 30-60 are reserved for use by Biochemistry 410 & 412 at Messiah College taught by Dr. Hannah Tims during Fall 2012 and Spring 2013. |
Contents |
Papain
Note: For some reason, using the "hydrogen bonds" link in the structure section breaks the jmol display and results in scenes after that point having random disulfide bonds and solvent molecules floating in mid-air. I've tried to fix it but to no avail, so if this happens just refresh the page and everything should work again. Oh look, now the new h-bond link in the substrate binding section does the same thing. If you see disembodied floating solvent molecules after clicking it, just refresh the page.
Overview
|
Papain is a 23.4 kDa, 212 residue cysteine endopeptidase originating from the fruit of Carica papaya, where it is present in significant amounts along with three other cysteine proteases, chymopapain, glycyl endopeptidase, and caricain[1][2][3]. Its action was first described by G.C. Roy in 1873. It was studied intensively from the 1950s to the 1960s, during which time it became the second enzyme ever to have its structure determined by x-ray crystallography. Finally, high resolution structural analysis in the 1980s allowed an accurate description of the enzyme's active site[3]. Papain displays very wide hydrolase activity, serving as a general amidase and esterase in addition to its protease activity. As a protease, papain can hydrolyze bonds of basic amino acids, leucine, and glycine. It shows preference for residues preceded by a large hydrophobic residue, but will not cleave if valine is present on the carboxyl side of a potential cleavage site. In addition to being a very non-specific enzyme, papain is also unusually heat resistant, with maximal activity occurring at a temperature of 65° C. These properties have led to use of papain in a large variety areas. One of these areas is biological research, where papain is utilized in cell isolation. It is also useful in immunological techniques because of its ability to cleave the connection between the crystallizable fragment domain and the immunoglobulin domain of antibodies[2]. Additionally, papain has found use outside of research as an inflammation control agent, a digestive aid, and even a meat tenderizer[4].
Structure
The secondary structure of papain consists of 7 , 17 , all of which are antiparallel, and a large amount (about 50% of total residues) of . The , which goes from blue (amino terminus) to red (carboxyl terminus) is useful for tracing the order of these structures through the chain. These secondary structures form as a result of favorable hydrogen bonding interactions within the polypeptide backbone. Meanwhile, secondary structures are kept in place by hydrophobic interactions and hydrogen bonds between sidechains of adjacent structures. For example, the (residues 25-42) is maintained as a result of between backbone carbonyl atoms and the hydrogen on the amide nitrogen four residues away. However, are present between this helix and the rest of the protein, suggesting that this helix is coordinated primarily by hydrophobic interactions. This is reasonable given its central location in the enzyme. As expected, the helix contains many (red residues are hydrophilic). The tertiary structure of papain is also maintained by three , which connect , , and [1]. These disulfide bonds are likely important in conserving the structural integrity of the enzyme as it operates in extracellular environments at high temperatures.
Residue Distribution
As can be seen from its , papain contains a variety of acidic (red), basic (blue), hydrophilic uncharged (pink), and hydrophobic (gray) residues. Since the enzyme operates in free solution, it would be expected that the hydrophilic residues would reside mostly on it exterior, shielding a hydrophobic core. This can be plainly seen from papain's , in which hydrophilic residues are pink and hydrophobic residues are orange. Upon removal of the hydrophilic residues, the of the enzyme is easy to see. As with all proteins, it is this hydrophobic segregation that allows the protein to properly fold and maintain a meaningful shape.
Ligands
The crystallization procedure used to obtain the 9PAP structure was carried out using a 62% (w/w) methanol and water crystallization medium. Thus, the papain in this crystal structure is coordinated by , 29 of which are shown, and , 21 of which occur between adjacent papain molecules and are probably important in maintaining their structural integrity[1]. Both (red and grey) and (purple) molecules form hydrogen bonds with many different residues, which are shown as ball and stick structures in the diagrams. Some of the ethanol molecules also have with the enzyme.
Catalytic Mechanism
|
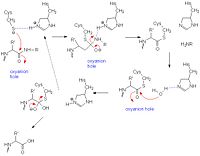
Like serine proteases, cysteine proteases contain a of residues. In the case of papain, these residues are Cys-25, His-159, and Arg-175. Papain also contains a fourth residue, that has been shown to play an important role in catalysis and is likely involved in the formation of the oxyanion hole. The mechanism begins when a peptide binds to the active site. Cys-25 is then deprotonated by His-159 and attacks the substrate carbonyl carbon. This forms a covalent, tetrahedral intermediate that is stabilized by the oxyanion hole. Next, His-159 acts as a general acid, protonating the nitrogen in the peptide bond, which acts as a leaving group as the carbonyl reforms. This now free C-terminal portion of the peptide is released. Water then enters the active site and attacks the carbonyl carbon while it is deprotonated by His-159, again forming an oxyanion hole-stabilized tetradral covalent intermediate. Finally, the carbonyl reforms and the Cys-25 sulfur acts as a leaving group, releasing the N-terminal portion of the peptide and regenerating the enzyme[6].
Substrate Binding
In order to investigate binding of protein substrates to papain, the enzyme was crystallized with the broad-spectrum competitive protease inhibitor leupeptin, shown in blue in the ribbon diagram. It has the structure Ac-Leu-Leu-Arginal, where Ac is an acetyl group attached to the nitrogen of the first leucine. The inhibitor functions by binding to the enzyme's active site, where the catalytic nucleophile (cysteine in papain) attacks the arginal aldehyde. This forms a tight-binding transition state from which the normal catalytic mechanism cannot proceed, due to this carbonyl having no potential leaving groups bonded to it. Analysis of the resulting structure revealed that the of papain consists primarily of a variety of , including tyrosine, tryptophan, and valine, which coordinate the bound leuptin. Some of the enzyme's residues also make with some of the leupeptin atoms. These hydrogen bonds, shown in yellow, include interactions between both hydrogens on both Gln-19 and the amide nitrogen of the catalytic Cys-25 with the arginal carbanion, forming the catalytically important oxyanion hole. In addition, Gly-66 interacts with the second leucine in leupeptin while Asp-158 interacts with a hydrogen on the arginal. These interaction further stabilize and orient the substrate in the binding pocket[7].
References
- ↑ 1.0 1.1 1.2 [1] 9PAP PDB
- ↑ 2.0 2.1 [2] Sigma Aldrich
- ↑ 3.0 3.1 [3] Worthington Biochemical Corporation
- ↑ [4] WebMD
- ↑ [5] University of Maine
- ↑ [6]Harrison, M.J., N.A. Burton, and I.H. Hillier. 1997. Catalytic Mechanism of the Enzyme Papain: Predictions with a Hybrid Quantum Mechanical/Molecular Mechanical Potential. J. Am. Chem. Soc. 119: 12285-12291
- ↑ [7] Schröder, E., C. Phillips, E. Garman, K. Harlos, C. Crawford. 1997. X-ray crystallographic structure of a papain-leupeptin complex. FEBS Letters 315: 38-42