User:Emma Ste.Marie/Sandbox 1
From Proteopedia
Contents |
Mammalian Thioredoxin Reductase (Type 1)
|
Introduction
Thioredoxin reductases (TrxRs) are a family of pyridine nucleotide-disulphide oxidoreductases that catalyze the reduction of thioredoxin, a class of small redox proteins known to be present in all organisms. Thioredoxin (Trx) plays a role in many important biological processes, including redox signalling. There are two classes of TRs that differ by size and the number of redox centers present in the enzyme. The lower molecular weight (Mr) TrxRs have two redox centers; a flavin adenine dinucleotide (FAD/H) and an N-terminal disulfide redox center[1]. Bacteria, plants, archaea and most unicellular eukaryotes utilize this low Mr, non-selenoprotein form of TrxR. In contrast, the mammalian-type TrxR (mTrxR) carries a C-terminal disulfide redox center in addition to the FAD/H and N-term disulfide redox centers. These higher Mr mTrxRs contain the selenium-containing amino acid selenocysteine (Sec)[1]. There are three human Sec-containing thioredoxin reductases: a cystolic form (type 1, mTrxR1), a mitochondrial form, and a specialized testes specific enzyme[2].
Disease
The Trx/mTrxR system is essential for maintaining redox homeostasis; there are numerous systems with thiol-dependent redox mechanisms, which are related to important pathological states and human diseases. Trx/mTrxR system plays a role in the following pathways: apoptosis, DNA replication and repair, protein repair, oxidative stress defence, redox signaling, cell cycle arrest, transcription, protein folding, and glucose metabolism. Disregulation of the Trx/mTrxR system is closely linked to many human diseases including: cardiovascular diseases, diabetes, cancer, aging, inflammation, virus infection, rheumatoid arthritis, and many others.
Because mTrxR1 depends so heavily on Sec498 to reduce Trx, diseases arise when the Sec residue is compromised. Sec can be bound by naturally or chemically occurring compounds rendering mTrxR1 inactive, leading to inactive Trx, and multiple diseases. Some natural compounds that inhibit mTrxR1 (bind near the catalytically important Sec residue) include: curcumin and curcumin analogs, quinones, flavonoids, HNE (4-hydroxy-2-nonenal) and other endogenous lipid electrophiles, and mercury and other metal ions[1].
Substrate Scope
mTrxRs have a wide substrate scope that arises from the fact that substrates may be reduced both by the easily accessible C-terminal selenolthiol motif of the NADPH-reduced enzyme, and some low-molecular weight substrates can also be reduced directly by the of the enzyme[1]. Lipoic acid, selenocystine, Ellman’s reagent, and hydrogen peroxide are a few small molecule substrates that can be reduced directly by the N-terminal redox active disulfide of mTrxRs[3]. Thioredoxin (Trx), a class of small (about 12kDa) redox active proteins, is the canonical substrate of mTrxRs. Trx plays a role in many important biological processes, including redox signalling[1, 4]. Trx is important for maintaining intracellular redox status as it is effective at scavenging hydrogen peroxide and hydroxyl radicals. Trxs contain two vicinal cysteines in a CXXC motif that are key to the ability of Trx to reduce other proteins. Upon reduction of other proteins, the vicinal Cys resdues of Trx are left in a disulfide that is subsequently reduced by mTrxR, returing Trx to its active form[1-2].
Structure
General: Three types of mTrxRs have been characterized: the cytosolic form (mTrxR1), the mitochondrial form (mTrxR2), and thioredoxin glutathione reductase (TGR) that is found in testes. TGR is a TrxR-glutathione reductase hybrid enzyme that possesses an N-terminal glutaredoxin-domain in addition to a C-terminal selenocysteine-containing redox centre[5]. Cytosolic mTrxR1s contain a C-terminal redox center with the sequence Xaa-Cys1-Cys2-Xaa (Cys-mTrxRs), or, Xaa-Cys-Sec-Xaa (Sec-mTrxRs)[6]. In contrast, mTrxR2s (the mitochondrial form), contain a C-terminal disulfide redox center with the sequence: Gly-Cys1-Gly-Gly-Gly-Lys-Cys2-Gly[6]. mTrxRs belong to a family of functional homodimeric pyridine nucleotide disulphide oxidoreductases with high homology to glutathione reductases (GRs) and lipoamide dehydrogenase (LipDH). The overall fold of mTrxR1 resembles other homodimeric pyridine nucleotide disulphide oxidoreductases[5]. mTrxRs function as head to tail homodimers, and each subunit contains a binding domain for FAD and NADPH, an interface domain, and a discrete active centre[6]. Currently, there are no crystal structures of the flexible C-terminal redox centre of mTrxR1 without substrate present. However, crystal structures of have been solved.
Motifs and Domains: Several Pfam domains (described by The European Bioinformatics Institute (EMBL-EBI)) present in mTrxR1 include: a pyridine nucleotide-disulphide oxidoreductase domain, pyridine nucleotide-disulphide oxidoreductase dimerisation domain, and nucleotide phosphate-binding region. Its substrate, thioredoxin, contains a glutaredoxin domain. Link to Pfam information on mTrxR1: [1] [2]
CATH database classifies mTrxR1 as an Alpha Beta protein, with 3-Layer(bba) Sandwich architecture, and a . It also contains an , and is considered a member of the Glutaredoxin homologous superfamily. Link to CATH information on mTrxR1: [3]
Interaction of mTrxR with Trx: The first crystal structure of Type 1 mTrxR in complex with its Trx substrate was solved by Karin Fritz-Wolf, et al. in 2011 and gave much information into structural details of the enzyme. The N-terminally located redox active disulfide () is buried in the protein, whereas the second, C-terminally located redox active selenosulfide (Cys497-Sec498) is positioned on a flexible, highly accessible C-terminal tail of the other subunit[5]. Complex formation of mTrxR1 with Trx involves the generation of an intermolecular disulphide bond between the catalytic residues which is stabilized by several other interactions. Some of the other interactions include: hydrophobic packing (mainly Trp resudies buried with their indole rings at the interface region), hydrogen bonds between amino acid side chain residues, and other interactions involving several oppositely charged residues (electrostatic interactions). All residues involved in forming the intermediate are highly conserved in mammals[5]. It is important to note in this crystal structure that penultimate Sec residues (one on each monomer) were mutated to Cys residues (E. coli, the expression vector used, does not have the machinery to incorporate Sec into proteins). Additionally, the resolving Cys residue was mutated to alanine to keep Trx bound.
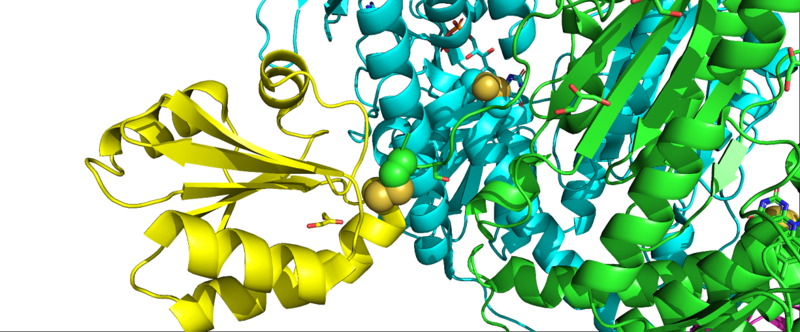
Role of Selenocysteine in Type 1 mTrxRs: Selenocysteine (Sec, U) is the 21st amino acid, and is structurally and chemically similar to cysteine (Cys, C). Unlike the other 20 amino acids, insertion of Sec into a protein is very complicated because a UGA stop codon must be recoded as a sense codon. Higher level eukaryotes posses special Sec-insertion machinery required for this process, such as a special stem-loop structure called a selenocysteine insertion sequence element (SECIS) in the 3’ untranslated region of the mRNA, special SECIS binding proteins, and selenocysteine elongation factors that bind specialized tRNA. The complexity of the process of inserting Sec into a protein signifies that Sec must fulfill a chemical function that conventional Cys residues cannot.
The role of Sec in mTrxR is not fully understood. One popular hypothesis is that Sec accelerates thiol disulfide exchange reactions in enzymes, thus conferring a kinetic advantage to Sec enzymes. Sec can accelerate thiol disulfide exchange reactions in three different ways [2, 6]. First, Sec can enhance the rate of the enzyme by being a better nucleophile than Cys (see figure). This is a reasonable hypothesis considering the lower pKa of Sec (pKa ~ 5.5) compared to Cys (pKa ~ 8.0); Sec would be deprotonated at a much lower pH (especially at physiological pH), making it a reactive nucleophile [2, 6]. Second, Sec is a better leaving group than Cys, and this accelerates the exchange reaction (see figure). Because the acidity of a selenol is ~1000 times greater than a thiol, selenols are superior leaving groups[2, 6]. Third, Sec is a better electrophile than Cys, and this greater electrophilicity accelerates the reaction (see figure). The greater tolerance for hypervalency of selenium has an important consequence, that nucleophilic attack on selenium (which typically forms or passes through hypervalent intermediates) usually occurs much more rapidly than at sulfur, since the intermediate selenium compounds are lower in energy than sulfur analogs[2, 6]. The Hondal research group has provided much evidence supporting the idea that mTrxR1 uses Sec as a superior electrophile[2, 7].

Another hypothesis for the role of Sec in mTrxR (and other seleno-enzymes) is that the Sec residue confers resistance to inactivation by oxidation to the enzyme. In 2013, G. Snider et al. showed that mTrxR1 is able to resist inactivation by oxidation from a variety of oxidants including hydrogen peroxide, hydroxyl radical, peroxynitrite, hypochlorous acid, hypobromous acid, and hypocyanous acid. A Cys-orthologue was also exposed to the oxidants, and the Cys- and Sec-enzymes were directly compared [8]. It was found that Sec-mTrxR is far superior to the Cys-orthologue TrxR in resisting inactivation by oxidation. The greatest divergence between selenium and sulfur chemistry occurs in the redox reactions of the two elements. Selenium is both a good nucleophile and a good electrophile, and this property allows it to easily cycle between reduced and oxidized states without becoming permanently oxidized [2,8]. These observations lead to the hypothesis that Sec confers a redox advantage to enzymes instead of a kinetic advantage.
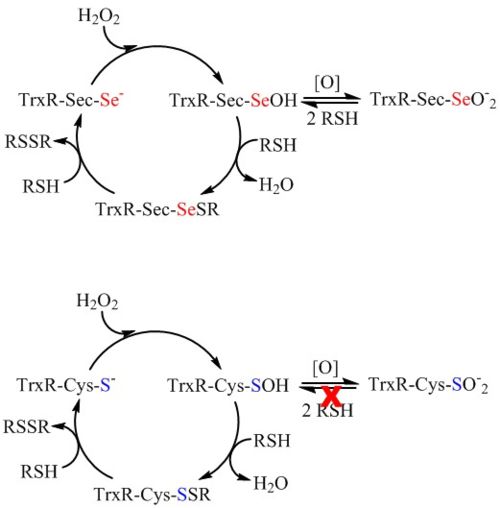
Analogues
Human TrxR1 and glutathione reductase (GR) share a high active site residue similarity at the cofactor and substrate binding sites as well as a similar reaction mechanism [5]. In Sec-mTrxRs, the 8 membered selenosulfide ring acts as an internal substrate that receives electrons from the N-terminal disulfide once it is reduced by NADPH. In contrast, GR is essentially a truncated form of mTrxR that do not contain the flexible C-terminal tail. Instead, GR reduces external substrates such as oxidized glutathione [6].
- Evolutionarily related proteins
- 3o0h - Glutathione reductase
- 1lvl - Dihydrolipoyl dehydrogenase
- 1ebd - Dihydrolipoamide dehydrogenase
- 1zk7 - Mercuric reductase (Hg(II) reductase)
- 3ef6 - Toluene 1,2-dioxygenase system ferredoxin
- 5jci - Putative cytosolic monodehydroascorbate reductase
- 1aog - Trypanothione reductase, putative
- 1fcd - Sulfide dehydrogenase, flavoprotein subunit
Mechanism
Sec-mTrxR works by using a hydride from NADPH to reduce a bound flavin. The reduced flavin on one subunit reduces the conserved N-terminal disulfide redox center (CICVNVGCCT), which in turn reduces the 8-membered selenosulfide ring of the Cys1-Sec2 dyad that is attached to the enzyme through a flexible peptide linker on the opposite subunit[5-6]. Once the C-terminal selenosulfide is reduced, it passes its electrons to the disulfide bond of Trx, forming a senosulfide bond with Trx. This mTrxR-Trx selenosulfide bond is then resolved by attack of Cys1 on Sec2. This results in the formation of a rare 8-membered selenosulfide ring. This ring is subsequently attacked and re-opened by attack of Se in Sec2 from CysIC.
3D Structures of Mammalian Thioredoxin Reductase
- mTrxR Type 1
Literature
- Crystal structure determination (PDB 3QFA): [4]
- Review articles:
- Related to disease:
- Biochemical and Biophysical Research Communications, 2010: [8]
References
[1] E. S. Arnér, Biochimica et Biophysica Acta (BBA)-General Subjects 2009, 1790, 495-526.
[2] H. J. Reich, R. J. Hondal, ACS chemical biology 2016, 11, 821-841.
[3] G. W. Snider, C. M. Dustin, E. L. Ruggles, R. J. Hondal, Biochemistry 2014, 53, 601-609; bA. P. Lothrop, E. L. Ruggles, R. J. Hondal, Biochemistry 2009, 48, 6213-6223.
[4] ScienceDirect, pp. From: The Chlamydomonas Sourcebook, 2009.
[5] K. Fritz-Wolf, S. Kehr, M. Stumpf, S. Rahlfs, K. Becker, Nature communications 2011, 2, 383.
[6] R. J. Hondal, E. L. Ruggles, Amino acids 2011, 41, 73-89.
[7] A. P. Lothrop, G. W. Snider, S. Flemer Jr, E. L. Ruggles, R. S. Davidson, A. L. Lamb, R. J. Hondal, Biochemistry 2014, 53, 664-674.
[8] G. W. Snider, E. L. Ruggles, N. Kahn, R. J. Hondal, Biochemistry 2013, 52, 5472-5481.
[9] CATH: Protein Structure Classification Database by I. Sillitoe, N. Dawson, T. Lewis, D. Lee, J. Lees, C. Orengo is licensed under a Creative Commons Attribution 4.0 International License.
Based on work at http://cath.biochem.ucl.ac.uk.
[10] B. Cunniff, G. W. Snider, N. Fredette, R. J. Hondal, N. H. Heintz, Analytical biochemistry 2013, 443, 34-40;
[11] D. Mustacich, G. Powis, Biochemical Journal 2000, 346, 1-8;
[12] T. Nauser, D. Steinmann, G. Grassi, W. H. Koppenol, Biochemistry 2014, 53, 5017-5022;
[13] E. L. Ruggles, P. B. Deker, R. J. Hondal, Journal of Peptide Science 2014, 20, 349-360;
[14] G. W. Snider, E. Ruggles, N. Khan, R. J. Hondal, Biochemistry 2013, 52, 5472-5481;
[15] D. M. Townsend, K. D. Tew, H. Tapiero, Biomedicine & Pharmacotherapy 2003, 57, 145-155.