Sandbox Reserved 895
From Proteopedia
This Sandbox is Reserved from Jan 13 through July 31, 2020 for use in the course Protein Structure in Drug Discovery taught by Karen C. Glass at the ACPHS, Colchester, United States. This reservation includes Sandbox Reserved 895 through Sandbox Reserved 901. |
To get started:
More help: Help:Editing |
Contents |
[1] Introduction
|
[1.1] The Retinoid (Visual) Cycle
For humans to see, the retinoid (visual) cycle converts incident light entering in the eye into electrochemical signals that can be transmitted to the brain as neuronal impulses. The chemical reactions that take place within the retinoid cycle allows the regeneration of key intermediates to allow for vision to take place. Dysregulation of these chemical processes along with gene mutations is associated with disease. These diseases often lead to an alteration in vision causing blindness. Age-related macular degeneration is an example of the dysregulation within the retinoid cycle. The 3-dimensional binding site of bovine RPE65 to an exogenous substrate (R)-emixustat shown in Figure 1A and Figure 1B. Figure 1C shows the surface of bovine RPE65 where (R)-emixustat would enter to access the internal binding pocket of RPE65. Both Figure 1B and Figure 1C was generated using PyMol. [2] (R)-emixustat is a drug candidate that was tested for the treatment of dry age-related macular degeneration.
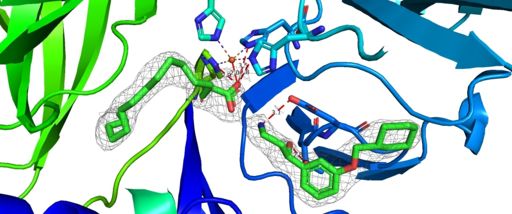
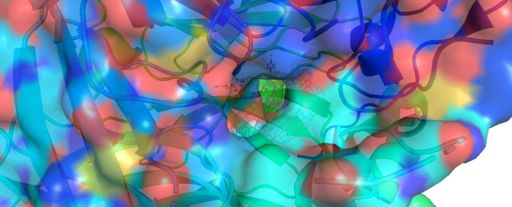
In order to stimulate the phototransduction pathway, 11-cis-retinal must be absorbed into the body. 11-cis-retinal often referred as Vitamin A, is an important dietary vitamin that starts the cascade of phototransduction. Once absorbed into the body and brought to the photoreceptor outer segment (POS), 11-cis-retinal is conjugated to opsin to form the rhodopsin complex. Upon light incidence, 11-cis-retinal is converted though a photo-isomerization reaction into all-trans-retinal. The conversion of 11-cis-retinal to all-trans-retinal causes a conformation change in the rhodopsin complex. This conformation change activates a G-protein coupled protein transducing which triggers the subsequent phototransduction cascade. The generated all-trans-retinal then dissociates from the rhodopsin complex which frees the opsin protein to bind another 11-cis-retinal molecule and restart the phototransduction cascade. All-trans-retinal must be converted back into 11-cis-retinal to serve as the chromophore for the phototransduction cascade. To regenerate 11-cis-retinal a series of enzyme catalyzed chemical reactions must take place. These reactions take place within the photoreceptor outer segment (POS) as well as the retinal pigment epithelium (hRPE).
Following dissociation from opsin, all-trans-retinal is transported from the lumen of the photoreceptor disk though an ATP-cassette transporter 4 (ABCA4). The enzyme all-trans-retinol dehydrogenase (atRDH) reduces all-trans-retinal into all-trans-retinol. Another transporter enzyme, interphotoreceptor retinoid-binding protein (IRBP) facilitates the transport of all-trans-retinol back into the hRPE. Within the hRPE, all-trans-retinol is esterified by the enzyme lecithin retinol acyltransferase (LRAT) to form all-trans-retinyl ester. RPE65 also known as retinoid isomerohydrolase (IMH) then converts all-trans-retinyl ester into 11-cis-retinol and palmityl acid. Following the RPE65 catalyzed reaction, 11-cis-retinol dehydrogenase (11-cis-RDH) oxidizes 11-cis-retinol into 11-cis-retinal. 11-cis-retinal is finally transported back into the photoreceptors to be conjugated to opsin to form the rhodopsin complex. This process is shown in Figure 2. [5]
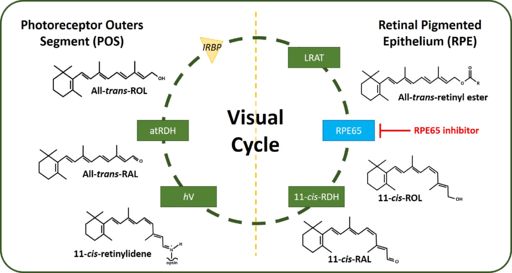
[1.2] RPE65
RPE65 is a known retinoid isomerohydrolase (IMH). In the visual cycle RPE65 catalyzes both an isomerization reaction which converts a trans double bond at carbon-11 to a cis configuration as well as a hydrolysis reaction that converts an ester functional group (the retinyl ester) into an alcohol functional group and a carboxylic acid using water. The catalyzed reaction is the rate limiting step in the retinoid cycle. Figure 3 shows the reaction schema. [7]
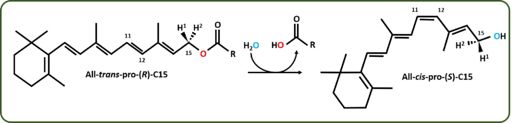
[1.2.1] Classification of RPE65
According to SCOP, the bovine RPE65 (PDB: 4RSC, SCOP: 8051041), belongs in the domain 8051041, the family 4007172 corresponding to retinoid isomerase RPE65-like, the superfamily 3002594 corresponding to RPE65-like, the fold 2001013 corresponding to 7-bladed beta-propeller and the class 100001 corresponding to all beta proteins. [9]
Using sequence homology, RPE65 belong to a family of proteins known as carotenoid cleavage oxygenase (CCO) enzymes. This class of enzymes often cleave β-carotene or apocarotenoids. However, what makes RPE65 unique from all the other enzymes in this family is that RPE65 catalyzes an isomerohydrolase reaction. Additionally, unlike the other enzymes in the CCO family, there is no obvious role for molecular oxygen in the RPE65 enzymology. All members of the CCO family contain four conserved histidine residues (His180, His241, His313 and His527) that bind to an ion (Fe2+) cofactor. [10] [11]
RPE65 can exist as both soluble and membrane bound forms which can undergo post-translational modifications (PTMs). At cysteine residues Cys231, Cys239 and Cys330 the enzyme can be S-palmitoylated. S-palmitoylation of RPE65 was speculated to increase the ratio of membrane-bound to soluble RPE65 which can increase the enzymatic activity. [12] However other studies have challenged this hypothesis and as such palmitoylation of RPE65 require further research to determine the activity. [13]
[1.2.2] Structural Analysis of RPE65
[1.2.2.1] Overall Structural Analysis of RPE65
Crystal structure of bovine RPE65 (PDB: 3FSN), which is 99% similar to human RPE65, (although the crystal structure for human RPE65 is not currently available) is used as the basis of studying RPE65 structure. RPE65 resembles a seven-bladed β-propeller with single-stranded extension on blades VI and VII and two-stranded extension on blade III, generated in PyMol and shown in Figure 4. The top face of the β-propeller is defined by connecting the outer strand of the β-sheet with the inner strand of the next β-sheet. The iron cofactor is located near the top surface of the propeller which is coordinated by four His residues and three secondary Glu residues. Each blade of the propeller contributes one His residue to coordinate with the iron cofactor. A hydrophobic tunnel leads the protein exterior to the active site which is defined by the iron ion to accommodate the passage of retinoids (which are conjugated to a fatty acid tail) from the membrane to the RPE65 catalytic site. The mouth of the tunnel is surrounded by three groups of nonpolar residues that contribute to the overall hydrophobicity of the tunnel and the integration with the lipid bilayer. There are also a few aromatic amino acid side chains that reside in this portion of the enzyme. This suggest that the depth of RPE65 membrane is restricted to the proximal portions of the phospholipid acyl chains with respect to the polar head groups. Arg and Lys residues within this region also contribute to the association with the negatively charged phospholipid head groups. [14]
[1.2.2.2] Active Site Structural Analysis of RPE65
Like stated before the RPE65 iron cofactor is found near the top surface of the β-propeller structure. The iron ion is directly coordinated by the Nitrogen-ε atoms of His180, His241, His313 and His527 with an average bond length of 2.2 angstroms. The geometry of the iron ion coordination is that of an octahedral with two open coordination sties. With the exception of His180, the other coordination interactions (His241, His313 and His527) is via hydrogen bonding from the Nδ-H bond on histidine to the Oε on Glu148, Glu417 and Glu469 respectively. The Nδ-H bond on His180 coordinates with a water molecule that is found lower axial, in the hydrophilic region of the propeller. These hydrogen bonds stabilize the octahedral coordination of the iron ion to facilitate the catalysis reaction. Of the two open coordination sties, one of the sites is occupied by the Cγ atom from Val134 located approximately 4.9 angstroms away. These interactions are shown in Figure 5.
As stated, the iron ion is also accessible through a main tunnel that enters the helical cap of the protein and is almost orthogonal relative to the propeller axis. This tunnel passes though the metal ion forming a bent cavity within the protein. A secondary tunnel within RPE65 leads from the exterior portion of the protein to the active site but contains a narrow segment that prevents the passage of retinoid substrates as well as other substrates catalyzed by RPE65. Although this second tunnel prevents larger compounds to enter, small molecules like water molecules or ions are allowed into the active site via this tunnel. As such it is hypothesized that the reactants and the products enter and exit from the same tunnel. The presence of amino acid residues such as Phe, Tyr and Trp within the main tunnel help confer enzyme rigidity as well as stabilize the intermediates of the isomerohydrolase reaction. The hydrophobicity of the cavity once again promotes the participation of lipophilic retinoids from the membrane into the active site as well as encourage the reaction of RPE65 with the membrane.
The main tunnel and its interior cavity contain two regions of strong residual electron density that is not accounted for by the atoms located in the protein. This suggest that the substrate would interact with these electron dense regions. The first electron dense region is linear with a triangular shape on one end, suggesting the presence of a linear molecule containing a terminal functional group with trigonal planar geometry (such as an ester in the retinyl esters or a carboxylic acid functional group in fatty acids). The triangular portion is in proximity to the iron ion and when positioned correctly fulfills one or both open coordination sites set forth by the octahedral geometry. The linear portion of the molecule then occupies the main tunnel. The second electron dense region is linear and bent in shape which is located within the interior cavity of the protein. This region could be represented by a bound PEG 200 molecule or a string of partially ordered water molecules. However, the electron dense region cannot accommodate longer compound such as (Hydroxyethyloxy)tri(ethyloxy)octane (C8E4).
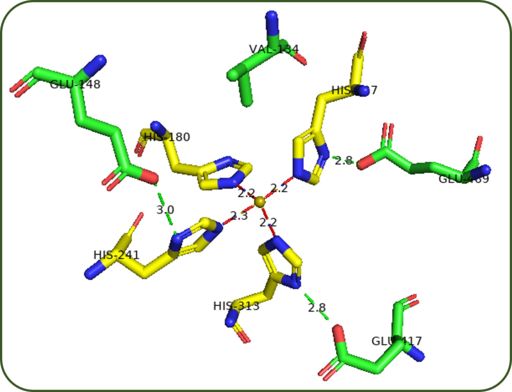
[1.2.3] Proposed Enzymatic Mechanism of RPE65
From an enzymatic point of view, RPE65 is similar to its carotenoid-cleaving relatives in that a ferrous ion is required for catalytic activity. Although the specific enzymatic mechanism for RPE65 is complex and not well elucidated, previous research has shown that the most probable reaction mechanism is shown in Figure 6A and Figure 6B. The initial interaction with the ester moiety of all-trans-retinyl ester with the ferrous ion polarizes the carbon-15 oxygen σ (sigma) bond and makes the fatty ester an activated leaving group. Formation of a resonance-stabilized carbocation reduces the π (pi) bond order of the system allowing temporary rotation about the carbon-11, carbon-12 σ bond. A water molecule obtained from the bulk solution attacks the carbon-15 atom quenching the carbocation intermediate. Finally, the protonation of the fatty acid carboxylate group promotes its dissociation from the ion cofactor. This process releases the fatty acid and the now 11-cis-retinol from the active site of RPE65. [19]
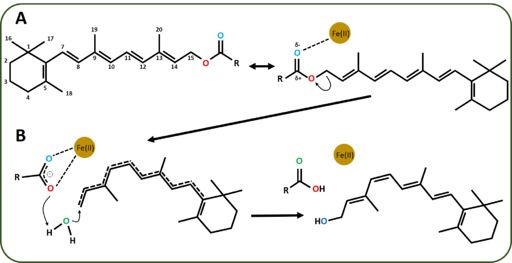
[1.3] Evolutionary Conservation
[1.3.1] Carotenoid Oxygenases
From an evolutionary standpoint it might be expected that the RPE65 catalytic mechanism would resemble that of the carotenoid oxygenase as sequence homology places RPE65 in the same family as the CCOs. This is not the case! Although the current enzymatic mechanism for RPE65 is only hypothesized with current research, there is not enough experimental evidence to definitively rule out that RPE65 catalyzes its function with molecular oxygen in the isomerization reaction. However, if such were the case the reaction would require chemistry that is even more complex which is not justifiable by the current scientific evidence. A study by Oberhauser and colleagues in 2008 on NinaB, a member of the CCO family from moths with isomerooxygenase activity was described, indicating that the members of this family originally developed isomerase activity while keeping the carotenoid oxygenase activity. The results of this study suggested a functional link between vertebrate RPE65 and insect NinaB which are both essential for the synthesis of key visual cycle chromophores. The function of NinaB showed that RPE65, a retinoid isomerase in the CCO family, retained the emergent activity that was found in a common ancestor. Comparing the topologies between the enzyme and substrate between NinaB and RPE65 showed that the iron cofactor was not directly involved in the double bond isomerization activity. Although further research is required to determine the ancestry of RPE65, it is nonetheless fascinating to study the evolution and the diversification of a protein family in animals by following the trace from a single multifunctional ancestral protein to several highly specialized enzymes seen in vertebrates. [21] Using Cluster Omega, the amino acid sequence of NinaB and bovine RPE65 was compared. [22] Although the structure alignment is quite different, there are still some sequence homology between the two enzymes that may suggest an ancestor linkage.
Comparing bovine RPE65 across different species using a BLAST search showed that RPE65 is highly conserved between animals. [23] From mammals, to rodents there was at least a 97% similarity in sequences. An example shown below in Figure 7A is a sequence alignment between bovine RPE65 and hamster RPE65. Throughout the sequence alignment there are only 12 amino acid residues that are different between the two species. By showing RPE65 is conserved, researchers are able to use bovine RPE65 to predict the structure and activity of human RPE65 in drug testing and drug development. Figure 7B shows the lineage of all know RPE65 enzymes.
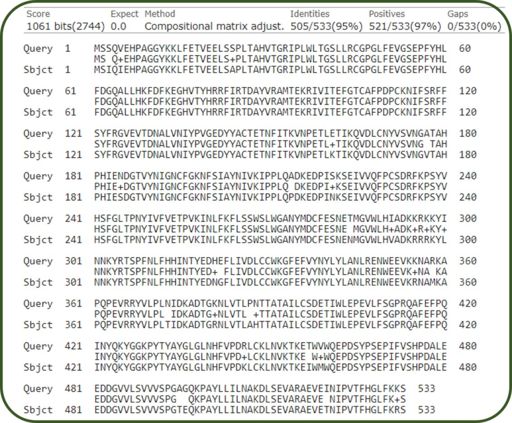
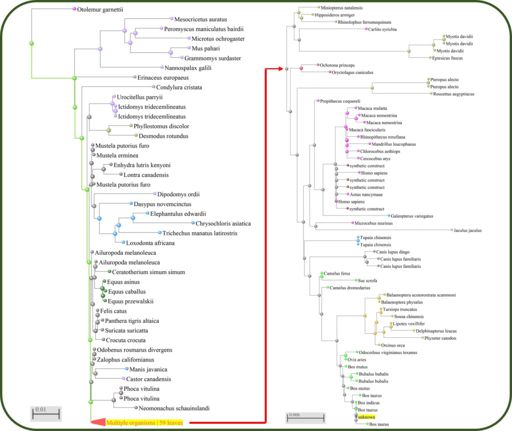
[1.3.2] Mutations leading to disease
Mutations within the RPE65 enzyme can impact the ability for the enzyme to catalyzed reactions that is pertinent towards the retinoid cycle. Disease such as Leber congenital amaurosis (LCA) or retinal dystrophy disease such as retinitis pigmentosa (RP) arise due to mutations in RPE65. Often these diseases can lead to impaired vision or even blindness. Since human and bovine RPE65 share approximately 99% of the amino acid sequences, it can be assumed that the secondary and tertiary structures are similar. This relationship allows us to map human LCA and RP to the bovine RPE65 amino acid sequences. In LCA and RP patients, it was noted that most of the amino acid mutations were adjacent to the blades of the β-propeller rather than in the connecting loops and helices. Blade VII was the most common area of mutation. Since mutations here would alter how the β-propeller seals into its proper structure, this could impact the location and residues that contribute to the coordination with the iron ion. For patients with LCA, missense mutations causes a major change in the first and second shell of the iron ion ligands which is essential for RPE65 isomerase activity. As such patients with these mutations had non-functional RPE65 enzymes. Amino acid Arg91, Tyr368 and His182 were most often mutated in patients with RPE65 associated LCA or RP. This showed that these amino acid positions are integral for enzymatic activity. Mutations such as His182Arg or Tyr368His caused major structural deformations that altered the substituted side chain. In such cases, enzyme activity was either decreased or lost. Arg91 is known to be important in positioning RPE65 to the membrane-binding elements. Arg91 also forms a salt bridge with Glu127 which is located on the C-terminal side of the enzyme. This interaction is critical for subcellular localization. As a result, mutations here alters the ability of RPE65 for subcellular localization. [26]
[2] Protein-Ligand Interaction
RPE65 can interact with other ligands to catalyze an enzymatic isomerohydrolase reaction.
[2.1] Endogenous Ligand, all-trans-retinyl ester
The endogenous ligand for human RPE65 is all-trans-retinyl ester. Binding to the active site as well as the catalytic mechanism were discussed above in the sections [1.2.2.2] Active Site Structural Analysis of RPE65 and [1.2.3] Proposed Enzymatic Mechanism of RPE65 respectively.
[2.2] Exogenous Ligand, emixustat
[2.2.1] (R)-emixustat (ACU-4429), Competitive Antagonist
[2.2.1.1] Development
Emixustat (ACU-4429) shown in Figure 8 is an investigational small molecule inhibitor of RPE65 first invented by a British-American chemist, Ian L. Scott. When synthesized, emixustat usually presents of a racemic mixture of (R)-emixustat and (S)-emixustat. The (R)-isomer is associated with increased binding affinity and potency and is used in drug development and research. Formulated as a hydrochloride salt, (R)-emixustat hydrochloride is taken by mouth and functions as a visual cycle modulator (VCM) in atrophic (dry) age-related macular degeneration (AMD). (R)-emixustat has been shown to reduce toxic retinal byproducts in the retinoid cycle such as N-retinylidiene-N-retinylethanolamine (A2E).
In 2008, Acucela Inc. partnered with Otsuka Pharmaceutical Company for the continued development of (R)-emixustat as a potential inhibitor of RPE65. Currently (R)-emixustat is in Phase III clinical trials in the United States for the potential treatment of Stargard's disease, a juvenile form of dry AMD. Additionally, (R)-emixustat is investigated as a potential therapy for diabetic retinopathy and diabetic macular edema. [27]
[2.2.1.2] Clinical Trials
Historically (R)-emixustat was tested as a possible treatment for dry AMD. In a Phase 1, dose-ranging clinical study of (R)-emixustat hydrochloride in healthy individuals the safety and tolerability of a 14-day course of either 5, 10, 20, 30 or 40 mg of (R)-emixustat was studied. A placebo in a 3 to 1 ratio was used. The results showed that (R)-emixustat was rapidly absorbed and very readily eliminated. Since the drug was rapidly eliminated, with a clearance of about 400% of that of hepatic blood flow, no significant accumulation of the drug was seen. Unfortunately, because of the high rate of elimination, the maximum concentration and the area under the curve was not significant from a clinical standpoint. Using the highest dose available, 40 mg, (R)-emixustat showed off-target effects and retinal toxicity liabilities. Using an electroretinogram, high dose (R)-emixustat inhibited the phototransduction process leading to blindness seen in LCA patients. As such (R)-emixustat was limited by both the minimum dose as well as the maximum dose. The use of (R)-emixustat in Phase IIb/III clinical trials failed to show clinical outcomes due to significant pharmacokinetic and pharmacodynamic limitations and thus was not approved by the FDA. Subsequent research is in progress to address the shortcomings of (R)-emixustat for the potential modulation of RPE65. [28]
[2.2.2] Structural Interaction and Stereoselectivity
Zhang and colleagues determined the binding interactions between RPE65 and emixustat by looking at the protein-ligand complex under three different considerations. The three considerations were RPE65 with a racemic emixustat, RPE65 with pure (R)-emixustat and RPE65 with pure (S)-emixustat. With all three cases, residual maps showed the presence of bound palmitate in the active site with the carboxylate oxygen forming a monodentate coordinate bond with the iron ion. The hydroxyl moiety in emixustat interacted via a hydrogen bond with the hydroxyl moiety in Thr147 whereas the primary amine was involved in a polar interaction with the carboxylate groups of Glu148 as well as the bound palmitate ligand. Crystal structure that was obtained in the presence of the racemic mixture of emixustat showed that the electron density was consistent with exclusive binding by the (R)-isomer (Figure 1B). Using pure (R)-isomer and the pure (S)-isomer as a follow up study to the experiment confirmed the stereoselectivity for the (R)-isomer of emixustat. Stereoselectivity was present because of the interaction between the hydroxyl moieties in the enantiomers. Looking at the electron density of the 3-amino-1-phenylpropan-1-ol moiety of (R)-emixustat showed that when the racemic mixture was used the binding was that of the (R)-isomer. This showed that (R)-emixustat had a higher binding affinity when compared to (S)-emixustat. The difference in binding affinity and potency was likely because of the less favorable polar interaction observed in the (S)-isomer. Bond lengths for the hydroxyl-Thr137 Oγ , amine-Glu148 Oε2 and amine-palmitate O1 interactions were 3.1, 3.2 and 2.8 angstroms for the (S)-isomer and 3, 2.7 and 2.6 angstroms for the (R)-isomer respectively (Figure 9).
The difference in bond lengths also confirms the increase in binding affinity and potency of the (R)-isomer. [29]
[3] Disease Implications, Medical Relevance and Current Drug Development
[3.1] Dry (atrophic) age related macular degeneration
Dry AMD represents the progressive neurodegenerative disorder which impact the specialize neurons within the central part of the retinal called the macula. The degeneration of choriocapillaires, hRPE and the neurosensory cells such as rods and cones photoreceptors can lead to sensory loss within the macula. Age-dependent accumulation of cytotoxic lipofuscin such as A2E within the hRPE can lead to irreversible damage in the retina. It is suggested that retinoid cycle modulation can help prevent the accumulation of lipofuscin in the hRPE. Since RPE65 is expressed exclusively within the hRPE cells, this makes RPE65 a potential for drug research and development as theoretically a drug targeting RPE65 would minimize off-target effects. RPE65 is responsible for the chemical conversion of all-trans-retinyl ester to 11-cis-retinol which is the rate limiting step, in the retinoid cycle. As such, modulating RPE65 would effectively modulate the entire retinoid cycle. [30]
Although (R)-emixustat failed to show clinical outcomes due to significant pharmacokinetic and pharmacodynamic limitations, (R)-emixustat became a proof-of-concept compound for RPE65 inhibition as well as the basis for structure-based drug design for future RPE65 inhibitors. Using (R)-emixustat, researchers were able to develop a screening for other RPE65 inhibitors. As part of the drug discovery process, subsequent hit identification, hit to lead, lead optimization processes would be required to develop a new line of RPE65 inhibitors. These processes would require the use of structure activity relationships as well as structural property relationships to overcome the shortfalls that emixustat presented.
[3.2] Stargardt’s Disease
Drastic accumulation of lipofuscin in the hRPE is a hallmark sign of juvenile-onset macular dystrophy also known as Stargardt’s Disease. It is suggested that retinoid cycle modulation can help prevent the drastic accumulation of lipofuscin leading to the development of Stargardt’s Disease. Currently in the United States, the use of (R)-emixustat is being tested in phase III clinical trails. [31]
[4] References
- ↑ Kiser PD, Golczak M, Lodowski DT, Chance MR, Palczewski K. Crystal structure of native RPE65, the retinoid isomerase of the visual cycle. Proc Natl Acad Sci U S A. 2009 Oct 13;106(41):17325-30. Epub 2009 Oct 5. PMID:19805034
- ↑ The PyMOL Molecular Graphics System, Version 2.3.3, Schrödinger, LLC.
- ↑ The PyMOL Molecular Graphics System, Version 2.3.3, Schrödinger, LLC.
- ↑ The PyMOL Molecular Graphics System, Version 2.3.3, Schrödinger, LLC.
- ↑ Shin Y, Moiseyev G, Petrukhin K, Cioffi CL, Muthuraman P, Takahashi Y, Ma JX. A novel RPE65 inhibitor CU239 suppresses visual cycle and prevents retinal degeneration. Biochim Biophys Acta Mol Basis Dis. 2018 Jul;1864(7):2420-2429. doi:, 10.1016/j.bbadis.2018.04.014. Epub 2018 Apr 21. PMID:29684583 doi:http://dx.doi.org/10.1016/j.bbadis.2018.04.014
- ↑ Shin Y, Moiseyev G, Petrukhin K, Cioffi CL, Muthuraman P, Takahashi Y, Ma JX. A novel RPE65 inhibitor CU239 suppresses visual cycle and prevents retinal degeneration. Biochim Biophys Acta Mol Basis Dis. 2018 Jul;1864(7):2420-2429. doi:, 10.1016/j.bbadis.2018.04.014. Epub 2018 Apr 21. PMID:29684583 doi:http://dx.doi.org/10.1016/j.bbadis.2018.04.014
- ↑ Kiser PD, Zhang J, Badiee M, Li Q, Shi W, Sui X, Golczak M, Tochtrop GP, Palczewski K. Catalytic mechanism of a retinoid isomerase essential for vertebrate vision. Nat Chem Biol. 2015 Jun;11(6):409-15. doi: 10.1038/nchembio.1799. Epub 2015 Apr, 20. PMID:25894083 doi:http://dx.doi.org/10.1038/nchembio.1799
- ↑ Kiser PD, Zhang J, Badiee M, Li Q, Shi W, Sui X, Golczak M, Tochtrop GP, Palczewski K. Catalytic mechanism of a retinoid isomerase essential for vertebrate vision. Nat Chem Biol. 2015 Jun;11(6):409-15. doi: 10.1038/nchembio.1799. Epub 2015 Apr, 20. PMID:25894083 doi:http://dx.doi.org/10.1038/nchembio.1799
- ↑ SCOP Databank 14 Apr 2020 Available from: http://scop.mrc-lmb.cam.ac.uk/term/8051041
- ↑ Kiser PD, Golczak M, Lodowski DT, Chance MR, Palczewski K. Crystal structure of native RPE65, the retinoid isomerase of the visual cycle. Proc Natl Acad Sci U S A. 2009 Oct 13;106(41):17325-30. Epub 2009 Oct 5. PMID:19805034
- ↑ Shin Y, Moiseyev G, Petrukhin K, Cioffi CL, Muthuraman P, Takahashi Y, Ma JX. A novel RPE65 inhibitor CU239 suppresses visual cycle and prevents retinal degeneration. Biochim Biophys Acta Mol Basis Dis. 2018 Jul;1864(7):2420-2429. doi:, 10.1016/j.bbadis.2018.04.014. Epub 2018 Apr 21. PMID:29684583 doi:http://dx.doi.org/10.1016/j.bbadis.2018.04.014
- ↑ Xue L, Gollapalli DR, Maiti P, Jahng WJ, Rando RR. A palmitoylation switch mechanism in the regulation of the visual cycle. Cell. 2004 Jun 11;117(6):761-71. PMID:15186777 doi:http://dx.doi.org/10.1016/j.cell.2004.05.016
- ↑ Jin M, Yuan Q, Li S, Travis GH. Role of LRAT on the retinoid isomerase activity and membrane association of Rpe65. J Biol Chem. 2007 Jul 20;282(29):20915-24. doi: 10.1074/jbc.M701432200. Epub 2007, May 15. PMID:17504753 doi:http://dx.doi.org/10.1074/jbc.M701432200
- ↑ Kiser PD, Golczak M, Lodowski DT, Chance MR, Palczewski K. Crystal structure of native RPE65, the retinoid isomerase of the visual cycle. Proc Natl Acad Sci U S A. 2009 Oct 13;106(41):17325-30. Epub 2009 Oct 5. PMID:19805034
- ↑ Kiser PD, Golczak M, Lodowski DT, Chance MR, Palczewski K. Crystal structure of native RPE65, the retinoid isomerase of the visual cycle. Proc Natl Acad Sci U S A. 2009 Oct 13;106(41):17325-30. Epub 2009 Oct 5. PMID:19805034
- ↑ The PyMOL Molecular Graphics System, Version 2.3.3, Schrödinger, LLC.
- ↑ Kiser PD, Golczak M, Lodowski DT, Chance MR, Palczewski K. Crystal structure of native RPE65, the retinoid isomerase of the visual cycle. Proc Natl Acad Sci U S A. 2009 Oct 13;106(41):17325-30. Epub 2009 Oct 5. PMID:19805034
- ↑ The PyMOL Molecular Graphics System, Version 2.3.3, Schrödinger, LLC.
- ↑ Kiser PD, Golczak M, Lodowski DT, Chance MR, Palczewski K. Crystal structure of native RPE65, the retinoid isomerase of the visual cycle. Proc Natl Acad Sci U S A. 2009 Oct 13;106(41):17325-30. Epub 2009 Oct 5. PMID:19805034
- ↑ Kiser PD, Golczak M, Lodowski DT, Chance MR, Palczewski K. Crystal structure of native RPE65, the retinoid isomerase of the visual cycle. Proc Natl Acad Sci U S A. 2009 Oct 13;106(41):17325-30. Epub 2009 Oct 5. PMID:19805034
- ↑ Oberhauser V, Voolstra O, Bangert A, von Lintig J, Vogt K. NinaB combines carotenoid oxygenase and retinoid isomerase activity in a single polypeptide. Proc Natl Acad Sci U S A. 2008 Dec 2;105(48):19000-5. doi:, 10.1073/pnas.0807805105. Epub 2008 Nov 19. PMID:19020100 doi:http://dx.doi.org/10.1073/pnas.0807805105
- ↑ Sievers F, Wilm A, Dineen D, Gibson TJ, Karplus K, Li W, Lopez R, McWilliam H, Remmert M, Soding J, Thompson JD, Higgins DG. Fast, scalable generation of high-quality protein multiple sequence alignments using Clustal Omega. Mol Syst Biol. 2011 Oct 11;7:539. doi: 10.1038/msb.2011.75. PMID:21988835 doi:http://dx.doi.org/10.1038/msb.2011.75
- ↑ Madden T. The BLAST Sequence Analysis Tool. 2002 Oct 9 [Updated 2003 Aug 13]. In: McEntyre J, Ostell J, editors. The NCBI Handbook [Internet]. Bethesda (MD): National Center for Biotechnology Information (US); 2002-. Chapter 16. Available from: http://www.ncbi.nlm.nih.gov/books/NBK21097/
- ↑ Madden T. The BLAST Sequence Analysis Tool. 2002 Oct 9 [Updated 2003 Aug 13]. In: McEntyre J, Ostell J, editors. The NCBI Handbook [Internet]. Bethesda (MD): National Center for Biotechnology Information (US); 2002-. Chapter 16. Available from: http://www.ncbi.nlm.nih.gov/books/NBK21097/
- ↑ Madden T. The BLAST Sequence Analysis Tool. 2002 Oct 9 [Updated 2003 Aug 13]. In: McEntyre J, Ostell J, editors. The NCBI Handbook [Internet]. Bethesda (MD): National Center for Biotechnology Information (US); 2002-. Chapter 16. Available from: http://www.ncbi.nlm.nih.gov/books/NBK21097/
- ↑ Kiser PD, Golczak M, Lodowski DT, Chance MR, Palczewski K. Crystal structure of native RPE65, the retinoid isomerase of the visual cycle. Proc Natl Acad Sci U S A. 2009 Oct 13;106(41):17325-30. Epub 2009 Oct 5. PMID:19805034
- ↑ Maekawa H. Acucela Provides Update on Emixustat Phase 3 Clinical Trial in Patients With Stargardt Disease. BioSpace. 13 Feb 2020. Available from: https://www.biospace.com/article/releases/acucela-provides-update-on-emixustat-phase-3-clinical-trial-in-patients-with-stargardt-disease/
- ↑ Kubota R, Al-Fayoumi S, Mallikaarjun S, Patil S, Bavik C, Chandler JW. Phase 1, dose-ranging study of emixustat hydrochloride (ACU-4429), a novel visual cycle modulator, in healthy volunteers. Retina. 2014 Mar;34(3):603-9. doi: 10.1097/01.iae.0000434565.80060.f8. PMID:24056528 doi:http://dx.doi.org/10.1097/01.iae.0000434565.80060.f8
- ↑ Zhang J, Kiser PD, Badiee M, Palczewska G, Dong Z, Golczak M, Tochtrop GP, Palczewski K. Molecular pharmacodynamics of emixustat in protection against retinal degeneration. J Clin Invest. 2015 Jul 1;125(7):2781-94. doi: 10.1172/JCI80950. Epub 2015 Jun, 15. PMID:26075817 doi:http://dx.doi.org/10.1172/JCI80950
- ↑ Hubschman JP, Reddy S, Schwartz SD. Age-related macular degeneration: current treatments. Clin Ophthalmol. 2009;3:155-66. doi: 10.2147/opth.s2094. Epub 2009 Jun 2. PMID:19668560 doi:http://dx.doi.org/10.2147/opth.s2094
- ↑ Kubota R, Al-Fayoumi S, Mallikaarjun S, Patil S, Bavik C, Chandler JW. Phase 1, dose-ranging study of emixustat hydrochloride (ACU-4429), a novel visual cycle modulator, in healthy volunteers. Retina. 2014 Mar;34(3):603-9. doi: 10.1097/01.iae.0000434565.80060.f8. PMID:24056528 doi:http://dx.doi.org/10.1097/01.iae.0000434565.80060.f8