Prp8
From Proteopedia
Contents |
Introduction
Prp8 Background
Prp8's name originates from the discovery that it is intricately involved with pre-m RNA processing within Eukaryotic organisms, and extensive research on the protein has revealed it is indispensable for the catalytic activity of the spliceosome earning it the nickname of 'Master Regulator of the Spliceosome' [1]. UV crosslinking experiments have shown that Prp8 has contact with the 5' splice site, 3' splice site, polypyrimidine tract, and branch point of pre-mRNA transcripts, as well as associating with all five of the snRNAs and their associated proteins [2]. Thus, Prp8 is the only protein to date involved with pre-mRNA splicing that actually contacts all catalytic elements of the spliceosome, indicating that it indeed has a very crucial role in pre-mRNA splicing catalysis, and resides in the catalytic heart of the splicing complex. Two other unique properties of Prp8 are its size and evolutionary conservation. Prp8 is the largest of all the spliceosomal proteins at approximately 250 kDa and is 2317 - 2416 amino acid residues in length (depending on the orthologue) [1]. In all organisms in which Prp8 has been identified Prp8, it is always been found as a U5 snRNP component and is highly conserved across species, with >60% identity between fungi, mammals, and plants [3]. Although Prp8 is highly conserved between species, its origin is shrouded in mystery because it contains no sequence homology to one family of proteins, but instead exhibits structural properties and domains from a range of conserved protein families while adding a twist to each that is Prp8 specific [1]. Even though Prp8 has been under extensive investigation for over a decade, its specific molecular mechanisms and functions are still unknown, but in light of all the information available, a hypothesis on Prp8's function can be postulated; Prp8 definitely acts as a large scaffold to coordinate the spliceosome's activity because the protein interacts with every part of the complex, however it is unclear whether Prp8 plays a direct role in splicing catalysis or only acts as a guide allowing for correct orientation and dynamic movements [1].
Pre-mRNA Splicing
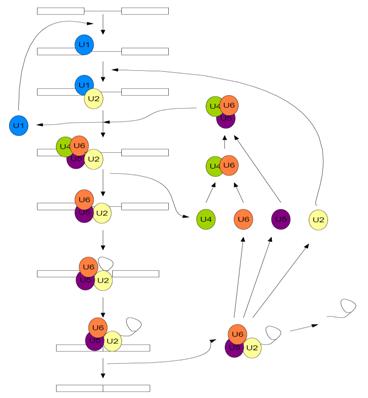
Pre-mRNA splicing is carried out by one of the most complex pieces of cellular machinery known to date; the Spliceosome. It is a large, catalytic protein-RNA complex responsible for removing the intronic sequences of pre-mRNA and “splicing” together the exonic sequences to form mature mRNA. The spliceosome is made up of approximately 145 distinct spliceosomal proteins, and five small nuclear RNAs (snRNAs) which each have a distinct set of proteins that interact with them forming snRNPs [5]. The five snRNAs have been subsequently named U1, U2, U4, U5, and U6. The spliceosome carries out a two-step transesterification reaction through a series of chemical steps in order to remove the intronic sequences from the pre-mRNA, which is facilitated by the movements, rearrangements, and dynamic exchanges of the snRNAs and associated splicing proteins [4].
First, the U1 snRNP recognizes and binds to the 5’ splice site of the pre-mRNA and the U2 snRNP recognizes and binds to the branch site of the intronic sequence, which contains a conserved adenine nucleotide, which is collectively known as complex A [6]. Subsequently, the U4/U6 U5 tri-snRNP moves in and associates with the intronic sequence (now known as complex B)[6]. This causes the U4 and U1 snRNPs to be displaced, forming the catalytically active spliceosome (complex B*) and the remaining U2, U5, and U6 snRNP facilitate the two transesterification reactions, dissociate from the now mature mRNA, and are recycled for subsequent use in another splicing reaction [6].
As stated above, the chemical mechanism pre-mRNA splicing follows is a two-step transesterification reaction. With the release of U1 and U4, following the subsequent rearrangement of the remaining snRNPs, the 2’ hydroxyl of the conserved adenine nucleotide carries out a nucleophilic attack on the 5’ splice site, which cuts the backbone of the mRNA, freeing the first exon [4]. Now the 5’ end of the intron is covalently bonded to the adenine nucleotide, forming a lariat structure [4]. Finally, the 3’ OH of the first exon nucleophilically attacks the 5’ end of the second exon, displacing the intron and splicing the exons together [4]. This is followed by the subsequent release of the lariat structure and the remaining spliceosome dissociates [4].
Structure of Prp8
| |||||||
'Prp8 C-terminus residues 1836 - 2397 3sbg | |||||||
---|---|---|---|---|---|---|---|
Gene: | DBF3, DNA39, PRP8, RNA8, SLT21, USA2, YHR165C (Saccharomyces cerevisiae) | ||||||
Related: | 3sbs, 3sbt
| ||||||
Resources: | FirstGlance, OCA, RCSB, PDBsum | ||||||
Coordinates: | save as pdb, mmCIF, xml |
Prp8's structure can be summarized as an amalgamation of domains from a variety of proteins, each of which has been slightly modified from their conventional forms and are now unique to Prp8. The major features present in Prp8 are a proline rich region, nuclear localization signal, bromodomain, reverse transcriptase-like domain, RNase H-like domain, and finally a metalloprotease/Jab1-like domain [7].
The proline rich region is present at the N-terminus of Prp8, approximately running from residues 5 - 78 [7]. This proline tract is found at the N-terminus of all fungal Prp8 sequences, and two rice sequences but is absent from most other organisms [1]. The proline rich region usually adopts an extended helical structure with three residues per turn [1]. The nuclear localization signal is also at the N-terminus of the protein, extending through amino acid residues 81 - 120, and can be located in the first 500 amino acids of the majority of organisms' Prp8 sequences [1]. The nuclear localization signal contains two clusters of positively charged amino acid residues which are separated by a variable region of 10 - 12 amino acids, and organisms lacking this classical signal motif usually possess an alternative within the N-terminus of their Prp8 [1]. The bromodomain is the last major region that is present at the N-terminus of Prp8, running from residues 200 - 315 [7]. It possesses low sequence identity (<14%) with commonplace bromodomains, but appears to contain no insertions or deletions despite its low identity with other sequences [7]. The domain consists of four alpha-helices and two loops which generally interact with and recognize lysine residues [7]. The recognition is facilitated by several highly conserved amino acids that stabilize the helix bundle [7].
The reverse transcriptase-like domain is located approximately halfway through Prp8 and extends through residues 950 - 1220 [7]. This domain is only reverse transcriptase-like because it does contain the conventional core set of alpha-helices and beta-strands that are a general characteristic of RT domains, but lacks key motifs that would normally confer its catalytic activity [7]. However, the Prp8 RT-like domain still possesses the potential to bind RNA [7]. Prp8 also contains an towards its C-terminal end (residues 1836 - 2092) [8]. The central region of the RNase H-like domain consists of a mixed alpha/beta fold, which resembles the overall shape of a mitten [8]. Following this analogy, the central 6 stranded beta sheets (yellow) and alpha helices (red) are the palm with a beta hairpin as the thumb and alpha helices as fingers which is all connected through regions of unstructured protein chain (dark blue). The groove created by the mitten like structure is lined with positive surface potential but also lacks key residues for RNase activity which is perfect for binding RNA, and has a very similar structure to the reverse transcriptase-like domain mentioned above [9]. The RNase domain possesses (in pink) at D1853/1781, D1854/1782, and Q1907/1835 (yeast/human numbering) which are thought to mediate interactions with the negatively charged backbone of an RNA molecule [9]. Finally, the last domain on the C-terminal end of Prp8 is a which extends through residues 2112 - 2413. Its overall structure consists of an oval shaped fold containing 12 beta strands (yellow), four alpha helices (red), and four 310 helices (red), which is all interconnected by an unstructured regions (dark blue) [10]. They form a mixed beta-barrel made up of seven strands surrounded by the remaining alpha and beta elements [10]. This domain also contains unique Prp8 residue substitutions; the commonplace metalloenzyme motif is replaced by which allows the domain to coordinate protein-protein contacts instead of a metal ion [10].
- Note - Thus far only C-terminal regions of Prp8 have been crystallized which is why only one PDB out of the half a dozen available is represented on this page.
Function of Prp8
Following suit with its unique structural makeup, Prp8 also possesses the potential to perform a variety of functions at the heart of the spliceosome. Above all else, Prp8 has been strongly implicated as a large scaffold which coordinates the active catalytic components of the spliceosome [1]. Prp8 acts as a cofactor for RNA splicing because it is required for the formation of the active (complex B*) spliceosome and for subsequent first and second transesterification reactions [1]. Prp8's bromodomain has been evolutionarily modified to recognize acetylated lysine residues, and given that many spliceosomal proteins are acetylated at lysine residues, it is likely that Prp8 mediates the assembly of spliceosome proteins and protein-RNA contacts through recognition of the lysine residues [3]. One example of such an interaction is Prp8's bromodomain mediated contact with Snu114p, which is a GTPase involved in RNA remodeling during the catalytic cycle of splicing [3].
Prp8's modified reverse transcritase and RNase H-like domains are postulated to function as recognition, interaction, and handover domains for snRNAs catalytically active in the spliceosome and portions of the pre-mRNA substrate [8]. Crosslinking experiments have shown that U5 and U6 snRNAs, as well as the GU dinucleotide of the 5' splice site, polypyrimidine tract (close to the intron branch point), and the 3' splice site all interact with or close to the reverse transcriptase and RNase H-like domains of Prp8 which supports the scaffolding and coordination role of Prp8 in pre-mRNA splicing [9][11][12]. As a result of Prp8's involvement with all the catalytic components of the spliceosome, the protein consequently plays a key role from start to finish in the splicing cycle; therefore, in sequence with the events of pre-mRNA splicing, Prp8 cooperates with Prp28 by bringing it into contact with the 5' splice site and coordinating Prp28's trans-helicase activity to handover the 5' splice site to U6 [9]. However, to complete the hand over and activation of the catalytic cycle the U4/U6 di-snRNP must be unwound. Prp8 also coordinates this event because Aar2p binds the RNase domain of Prp8 which sequesters the MPN/Jab1 domain, however when Aar2p is phosphorylated, Brr2 is able to interact withe the released C-terminus, which activates Brr2's helicase activity allowing for the unwinding of U4 and U6, and subsequently the 5' splice site handover and catalytic activation of the first step of pre-mRNA splicing [8]. The catalytic activity of the spliceosome is also potentially coordinated by Prp8 which is explained by the 'lock and load' hypothesis which is as follows; following the 5' splice site activation, the first transesterification step is carried out, and Prp8 subsequently locks the 5' splice site into position and loads the 3' splice site into position for catalysis of the second transesterification step and subsequent completion of the splicing cycle [1]. This is all thought to be facilitated by Prp8's modified domains that don't retain any of their rudimentary catalytic functions, and are instead specialized only for RNA/protein binding and coordination. However, the molecular specifics of Prp8's key role in the catalysis of pre-mRNA splicing are still largely unknown.
Evolution of Prp8
The fact that Prp8 contains so many unique structural features has also raised the question of how the integral splicing protein evolved. Domain dissection of the protein has revealed that the whole central portion (residues 770-2173) can function as a discrete unit [3]. As it turns out, almost all of the protein and RNA contacts, as well as the unique domains of Prp8, are contained within this region. This obviously leads to the hypothesis that the central portion referred to above may have been the original Prp8 protein [3]. It follows that over the course of evolution, the protein has become modified and attained its N and C-terminal regions (including the bromodomain and MPN/Jab1 domain)[3]. One reason why these regions may have become so highly conserved is to retain the additional ability for protein contacts within the spliceosome [3]. This would confer a selective advantage because it allows Prp8 to coordinate and correctly position additional key spliceosomal components which may have been gained for increased control and precision of the spliceosome in higher Eukaryotes [3]. However, like the specific molecular mechanisms conferred by Prp8, its evolutionary tale remains a mystery thus far [3].
References
- ↑ 1.00 1.01 1.02 1.03 1.04 1.05 1.06 1.07 1.08 1.09 1.10 Grainger RJ, Beggs JD. Prp8 protein: at the heart of the spliceosome. RNA. 2005 May;11(5):533-57. PMID:15840809 doi:10.1261/rna.2220705
- ↑ Siatecka M, Reyes JL, Konarska MM. Functional interactions of Prp8 with both splice sites at the spliceosomal catalytic center. Genes Dev. 1999 Aug 1;13(15):1983-93. PMID:10444596
- ↑ 3.0 3.1 3.2 3.3 3.4 3.5 3.6 3.7 3.8 Dlakic M, Mushegian A. Prp8, the pivotal protein of the spliceosomal catalytic center, evolved from a retroelement-encoded reverse transcriptase. RNA. 2011 May;17(5):799-808. Epub 2011 Mar 25. PMID:21441348 doi:10.1261/rna.2396011
- ↑ 4.0 4.1 4.2 4.3 4.4 4.5 Guthrie C. Messenger RNA splicing in yeast: clues to why the spliceosome is a ribonucleoprotein. Science. 1991 Jul 12;253(5016):157-63. PMID:1853200
- ↑ Zhou Z, Licklider LJ, Gygi SP, Reed R. Comprehensive proteomic analysis of the human spliceosome. Nature. 2002 Sep 12;419(6903):182-5. PMID:12226669 doi:10.1038/nature01031
- ↑ 6.0 6.1 6.2 Green MR. Biochemical mechanisms of constitutive and regulated pre-mRNA splicing. Annu Rev Cell Biol. 1991;7:559-99. PMID:1839712 doi:http://dx.doi.org/10.1146/annurev.cb.07.110191.003015
- ↑ 7.0 7.1 7.2 7.3 7.4 7.5 7.6 7.7 7.8 Dlakic M, Mushegian A. Prp8, the pivotal protein of the spliceosomal catalytic center, evolved from a retroelement-encoded reverse transcriptase. RNA. 2011 May;17(5):799-808. Epub 2011 Mar 25. PMID:21441348 doi:10.1261/rna.2396011
- ↑ 8.0 8.1 8.2 8.3 Weber G, Cristao VF, Alves FD, Santos KF, Holton N, Rappsilber J, Beggs JD, Wahl MC. Mechanism for Aar2p function as a U5 snRNP assembly factor. Genes Dev. 2011 Jul 15. PMID:21764848 doi:10.1101/gad.635911
- ↑ 9.0 9.1 9.2 9.3 Pena V, Rozov A, Fabrizio P, Luhrmann R, Wahl MC. Structure and function of an RNase H domain at the heart of the spliceosome. EMBO J. 2008 Nov 5;27(21):2929-40. Epub 2008 Oct 9. PMID:18843295 doi:10.1038/emboj.2008.209
- ↑ 10.0 10.1 10.2 Pena V, Liu S, Bujnicki JM, Luhrmann R, Wahl MC. Structure of a multipartite protein-protein interaction domain in splicing factor prp8 and its link to retinitis pigmentosa. Mol Cell. 2007 Feb 23;25(4):615-24. PMID:17317632 doi:10.1016/j.molcel.2007.01.023
- ↑ Kuhn AN, Li Z, Brow DA. Splicing factor Prp8 governs U4/U6 RNA unwinding during activation of the spliceosome. Mol Cell. 1999 Jan;3(1):65-75. PMID:10024880
- ↑ Brown JD, Beggs JD. Roles of PRP8 protein in the assembly of splicing complexes. EMBO J. 1992 Oct;11(10):3721-9. PMID:1396567