User:David Jung/BCHM3981 RTP Tus
From Proteopedia
The Replication Terminator Protein (RTP) is a protein involved in termination of replication in the gram positive bacterium, Bacillus subtilis. RTP was first identified in 1989, showing analogous function to Tus protein present in Escherichia coli (Lewis et al. 1989). Both RTP and Tus bind to termination sites (Ter sequences) present in the bacterial chromosome, terminating replication. Polar directionality of termination is assumed as circular bacterial chromosome is replicated bidirectionally with one replication fork going clockwise and the other one going anticlockwise. The RTP-Ter complex must therefore allow the replication fork to pass unimpeded from the “permissive” direction, while halting the fork from the “blocking” direction (Griffiths, Andersen, and Wake, 1998). This is demonstrated in the Escherichia coli counterpart due to its apparent asymmetric structure (Kamada et al., 1996). However, this asymmetry is not observed in RTP's structure.
Even though the protein is known to be involved in replication termination, its biological function is not well understood as mutants that lack certain Ter sites are shown to be viable in vitro (Iismaa and Wake. 1987). Thus, the biological functions of replication terminator proteins in bacteria have long been speculated. There are two hypotheses: inhibition of production of multimeric DNA, and post-initiation control of replication. Multimeric DNA is a dsDNA where multiple copies of the whole sequence are present. It has been shown, in the case of Tus in Escherichia coli, that without Tus-Ter interaction, it is more prone to overreplication (Hiasa and Marians, 1994). This is thought to be due to lack of inhibition of movement of DnaB, a helicase, along the replication fork by Tus-Ter complex. It was also shown that replication terminator protein is involved in post-initiation control of replication. The first level of control of replication was thought to occur before initiation. However, it has been experimentally determined that RTP-Ter complex maybe involved in second level of control after initiation to inhibit overreplication (Henckes et al., 1989).
Contents |
Ter sites
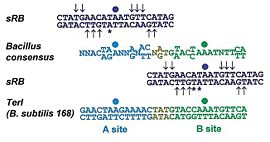
B. subtilis possesses 9 Ter sites (TerI – IX), 4 able to terminate the clockwise replication fork and 5 able to terminate the anticlockwise replication fork (Fig 1). Each Ter sites are 30 bp sequences consisting of two 16-17 bp imperfect inverted repeats called the A site and B site that overlap at a fairly conserved trinucleotide sequence (Lewis et al., 1990) (Fig 2). Each A and B half-site binds a dimer of RTP and fork arrest only occurs when the fork approaches from the B site end (Smith and Wake, 1992).
Structure
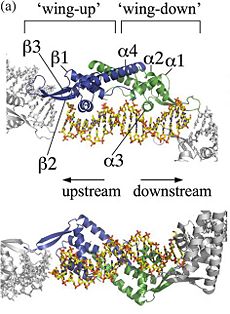
|
The classical winged-helix motif consists of an αβααββ topology where the “wing” is a loop between the β2-β3 strands. Each RTP monomer comprises of a modified winged-helix motif. It has the β1-strand replaced by a loop and an additional α4 helix following the β3-strand (Vivian et al., 2007) (Fig 3). The α4 helix acts as a dimerisation interface, forming an antiparallel coiled coil with α4 helix of the other monomer (Vivian et al., 2007). The α3 helix is the recognition helix and is involved in the specific binding with the DNA sequences via the major groove (Vivian et al., 2007) (Fig 3, 4). The wings are involved in both protein:proteins and protein:DNA contacts (Manna et al., 1996b; Pai et al., 1996). Initially, the RTP dimer was considered to be symmetrical, however this was due the the symmetrical nature of the artificial Ter sequence (sRB) used in the study (Wilce et al., 2001) (Fig 2). Later, Vivian et al. was able to demonstrate that the RTP dimer is in fact slightly asymmetrical when bound to the native RTP TerI B site (nRB) (Fig 5a) with the greatest deviation in the β1-loop and the wing regions (Fig 5b) (Vivian et al., 2007). Thus the monomers were differentiated as “wing-up” (upstream) and “wing-down” (downstream) (Fig 3).
|
|
|
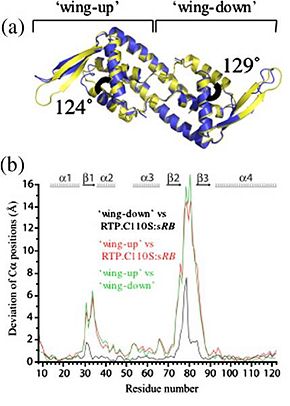
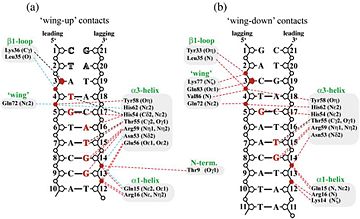
Mechanism
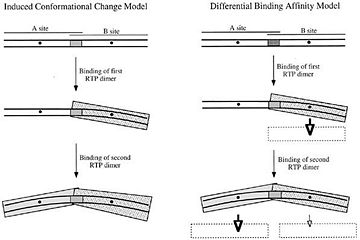
It appears that cooperative binding at both A and B sites are required for fork arrest. It has been demonstrated that binding at B site is a pre-requisite to A site binding (Langley et al., 1993) and binding of B site alone on a vector is not sufficient to stop the advancing helicase (Smith et al., 1996; Smith et al., 1994; Smith and Wake, 1992). Only when both half-sites are filled (KD = 3 x 10-11 M) that fork arrest occurs (Duggin et al., 1999). Many hypotheses and models have been proposed by a number of researchers to explain this polar mechanism for replication termination (Duggin et al., 2005; Kralicek et al., 1997; Manna et al., 1996a; Manna et al., 1996b; Wake, 1997). Overall, there have been two models: the differential binding affinity model and the induced conformational change model, both proposed by Kralicek et al. in 1997 (Kralicek et al., 1997).
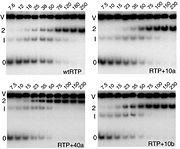
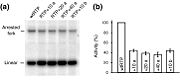
Differential binding affinity model
Differential binding affinity (aka the “directional molecular clamp” in E. coli (Lee and Kornberg, 1992; Lee et al., 1989; Mohanty, Sahoo, and Bastia, 1998; Sahoo et al., 1995)) model was proposed with the claim that the polarity is induced by binding of RTP dimers to each site with different strengths (Fig 8). It was hypothesised that the RTP dimer binding to the half site located in the "blocking" site binds tightly while the dimer binding to the "permissive" site binds less tightly to the site (Langley et al., 1993). Duggin et al. carried out a series of experiments which supported cooperative binding. When a complete TerI sequence had its nRB site substituted with sRB, there was a fivefold reduction in the cooperative binding of the A site and a 50% reduction in fork arrest. Further substitutions of A and B sites with sRB found that there mutant sequences resulted in reduction in fork arrest despite similar binding affinities (Duggin et al., 2005). Furthermore, Duggin experimented with RTP fusion proteins consisting of extra peptides (GFP, 10, 20 or 40 residues long) attached to the C-terminus of α4-helix which was predicted to contact the approaching replisome. In vivo, these fusion proteins showed normal RTP-DNA binding affinity on the gel shift assay, but Southern Blot showed that replication fork arrest efficiency was reduced, not directly proportional to peptide size or sequence (Duggin, 2006) (Fig 6, 7). These experiments suggest that differential binding affinity cannot be the sole explanation for the polar fork arrest. However Duggin’s experiment also implies that specific interactions of RTP α4-helix with helicase is not the sole contributor in fork arrest either, as ~40% fork arrest activity was still present despite fusion peptide interference (Fig 7b).
Induced conformational change model
Kralicek et al. proposed that polar fork arrest arises as a consequence of the two RTP-DNA half-sites having different conformations; which result from different RTP-DNA contacts at the asymmetrical half-sites, as well as interactions between the RTP dimers on the DNA (Kralicek et al., 1997) (Fig 8). The sequential binding of two RTP dimers to each half-site bends and underwinds the DNA additively (Kralicek et al., 1997). In Vivian et al.’s X-ray crystallography work on RTP.C110S: nRB complexes, they observed underwinding of the B site’s upstream end, which suggests that the upstream wing may be involved in contacting the A site to support bending of the DNA (Vivian et al., 2007). The bending of DNA is thought to facilitate the two RTP dimer’s interactions. Mutagenesis have shown that residues on the β3-strand and β1 loop are crucial in cooperative binding at the A site (Manna et al., 1996b).
References
Duggin, I. G. (2006). DNA replication fork arrest by the Bacillus subtilis RTP-DNA complex involves a mechanism that is independent of the affinity of RTP-DNA binding. Journal of Molecular Biology 361(1), 1-6.
Duggin, I. G., Andersen, P. A., Smith, M. T., Wilce, J. A., King, G. F., and Wake, R. G. (1999). Site-directed mutants of RTP of Bacillus subtilis and the mechanism of replication fork arrest. Journal of Molecular Biology 286(5), 1325-1335.
Duggin, I. G., Matthews, J. M., Dixon, N. E., Wake, R. G., and Mackay, J. P. (2005). A complex mechanism determines polarity of DNA replication fork arrest by the replication terminator complex of Bacillus subtilis. Journal of Biological Chemistry 280(13), 13105-13113.
Griffiths, A. A., Andersen, P. A., and Wake, R. G. (1998). Replication terminator protein-based replication fork-arrest systems in various Bacillus species. Journal of Bacteriology 180(13), 3360-3367.
Kamada, K., Horiuchi, T., Ohsumi, K., Shimamoto, N., and Morikawa, K. (1996). Structure of a replication-terminator protein complexed with DNA. Nature 383(6601), 598-603.
Kralicek, A. V., Wilson, P. K., Ralston, G. B., Wake, R. G., and King, G. F. (1997). Reorganization of terminator DNA upon binding replication terminator protein: Implications for the functional replication fork arrest complex. Nucleic Acids Research 25(3), 590-596.
Langley, D. B., Smith, M. T., Lewis, P. J., and Wake, R. G. (1993). Protein-nucleoside contacts in the interaction between the replication terminator protein of bacillus-subtilis and the DNA terminator. Molecular Microbiology 10(4), 771-779.
Lee, E. H., and Kornberg, A. (1992). Features of replication fork blockage by the Escherichia coli terminus binding protein. Journal of Biological Chemistry 267(13), 8778-8784.
Lee, E. H., Kornberg, A., Hidaka, M., Kobayashi, T., and Horiuchi, T. (1989). Escherichia coli replication termination protein impedes the action of helicases. Proceedings of the National Academy of Sciences of the United States of America 86(23), 9104-9108.
Lewis, P. J., Ralston, G. B., Christopherson, R. I., and Wake, R. G. (1990). Identification of the replication terminator protein-binding sites in the terminus region of the Bacillus subtilis chromosome and stoichiometry of the binding. Journal of Molecular Biology 214(1), 73-84.
Lewis, P.J., Smith, M.T. and Wake, R.G. (1989). A protein involved in termination of chromosome replication in Bacillus subtilis binds specifically to the terC site. J. Bacteriology. 171(6):3564-3567.
Manna, A. C., Pai, K. S., Bussiere, D. E., Davies, C., White, S. W., and Bastia, D. (1996a). Helicase-contrahelicase interaction and the mechanism of termination of DNA replication. Cell 87(5), 881-891.
Manna, A. C., Pai, K. S., Bussiere, D. E., White, S. W., and Bastia, D. (1996b). The dimer-dimer interaction surface of the replication terminator protein of Bacillus subtilis and termination of DNA replication. Proceedings of the National Academy of Sciences of the United States of America 93(8), 3253-3258.
Mohanty, B. K., Sahoo, T., and Bastia, D. (1998). Mechanistic studies on the impact of transcription on sequence-specific termination of DNA replication and vice versa. Journal of Biological Chemistry 273(5), 3051-3059.
Pai, K. S., Bussiere, D. E., Wang, F. G., Hutchison, C. A., White, S. W., and Bastia, D. (1996). The structure and function of the replication terminator protein of Bacillus subtilis: Identification of the 'winged helix' DNA-binding domain. Embo Journal 15(12), 3164-3173.
Sahoo, T., Mohanty, B. K., Lobert, M., Manna, A. C., and Bastia, D. (1995). The contrahelicase activities of the replication terminator proteins of Escherichia coli and Bacillus subtilis are helicase-specific and impede both helicase translocation and authentic DNA unwinding. Journal of Biological Chemistry 270(49), 29138-29144.
Smith, M. T., deVries, C. J., Langley, D. B., King, G. F., and Wake, R. G. (1996). The Bacillus subtilis DNA replication terminator. Journal of Molecular Biology 260(1), 54-69.
Smith, M. T., Langley, D. B., Young, P. A., and Wake, R. G. (1994). The minimal sequence needed to define a functional dna terminator in Bacillus subtilis. Journal of Molecular Biology 241(3), 335-340.
Smith, M. T., and Wake, R. G. (1992). Definition and polarity of action of DNA replication terminators in Bacillus subtilis. Journal of Molecular Biology 227(3), 648-657.
Vivian, J. P., Porter, C. J., Wilce, J. A., and Wilce, M. C. J. (2007). An asymmetric structure of the Bacillus subtilis replication terminator protein in complex with DNA. Journal of Molecular Biology 370(3), 481-491.
Wake, R. G. (1997). Replication fork arrest and termination of chromosome replication in Bacillus subtilis. Fems Microbiology Letters 153(2), 247-254.
Wilce, J. A., Vivian, J. P., Hastings, A. F., Otting, G., Folmer, R. H. A., Duggin, I. G., Wake, R. G., and Wilce, M. C. J. (2001). Structure of the RTP-DNA complex and the mechanism of polar replication fork arrest. Nature Structural Biology 8(3), 206-210.