User:Michael Patrick/Sandbox 2
From Proteopedia
Contents |
GREEN FLUORESCENT PROTEIN
INTRODUCTION
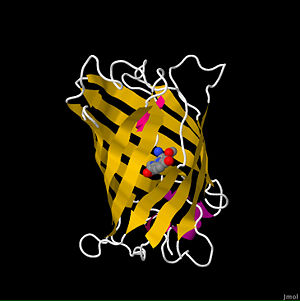
The green fluorescent protein (GFP) is a protein composed of 238 amino acid residues (26.9kDa) that exhibits bright green fluorescence when exposed to blue light. Although many other marine organisms have similar green fluorescent proteins, GFP traditionally refers to the protein first isolated from the jellyfish Aequorea victoria. The GFP from A. victoria has a major excitation peak at a wavelength of 395 nm and a minor one at 475 nm. Its emission peak is at 509 nm, which is in the lower green portion of the visible spectrum. The GFP from the sea pansy (Renilla reniformis) has a single major excitation peak at 498 nm. In cell and molecular biology, the GFP gene is frequently used as a reporter of expression. In modified forms it has been used to make biosensors, and many animals have been created that express GFP as a proof-of-concept that a gene can be expressed throughout a given organism. The GFP gene can be introduced into organisms and maintained in their genome through breeding, injection with a viral vector, or cell transformation. To date, the GFP gene has been introduced and expressed in many bacteria, yeast and other fungi, fish (such as zebrafish), plant, fly, and mammalian cells, including human. Martin Chalfie, Osamu Shimomura, and Roger Y. Tsien were awarded the 2008 Nobel Prize in Chemistry on 10 October 2008 for their discovery and development of the green fluorescent protein.
HISTORY
In the 1960s and 1970s, GFP, along with the separate luminescent protein aequorin, was first purified from Aequorea victoria and its properties studied by Osamu Shimomura.[1] In A. victoria, GFP fluorescence occurs when aequorin interacts with Ca2+ ions, inducing a blue glow. Some of this luminescent energy is transferred to the GFP, shifting the overall color towards green.[2] However, its utility as a tool for molecular biologists did not begin to be realized until 1992 when Douglas Prasher reported the cloning and nucleotide sequence of wtGFP in Gene.[3] The funding for this project had run out, so Prasher sent cDNA samples to several labs. The lab of Martin Chalfie expressed the coding sequence of wtGFP, with the first few amino acids deleted, in heterologous cells of E. coli and C. elegans, publishing the results in Science in 1994.[4] Frederick Tsuji's lab independently reported the expression of the recombinant protein one month later.[5] Remarkably, the GFP molecule folded and was fluorescent at room temperature, without the need for exogenous cofactors specific to the jellyfish. Although this near-wtGFP was fluorescent, it had several drawbacks, including dual peaked excitation spectra, pH sensitivity, chloride sensitivity, poor fluorescence quantum yield, poor photostability and poor folding at 37°C.
The first reported crystal structure of a GFP was that of the S65T mutant by the Remington group in Science in 1996.[5] One month later, the Phillips group independently reported the wild-type GFP structure in Nature Biotech.[9] These crystal structures provided vital background on chromophore formation and neighboring residue interactions. Researchers have modified these residues by directed and random mutagenesis to produce the wide variety of GFP derivatives in use today.
GFP DERIVATIVES
Due to the potential for widespread usage and the evolving needs of researchers, many different mutants of GFP have been engineered. The first major improvement was a single point mutation (S65T) reported in 1995 in Nature by Roger Tsien[10]. This mutation dramatically improved the spectral characteristics of GFP, resulting in increased fluorescence, photostability, and a shift of the major excitation peak to 488 nm, with the peak emission kept at 509 nm. This matched the spectral characteristics of commonly available FITC filter sets, increasing the practicality of use by the general researcher. A 37 °C folding efficiency (F64L) point mutant to this scaffold yielding enhanced GFP (EGFP) was discovered in 1995 by the lab of Ole Thastrup[11] EGFP allowed the practical use of GFPs in mammalian cells. EGFP has an extinction coefficient (denoted ε) of 55,000 M−1cm−1. The fluorescence quantum yield (QY) of EGFP is 0.60. The relative brightness, expressed as ε•QY, is 33,000 M−1cm−1. Superfolder GFP, a series of mutations that allow GFP to rapidly fold and mature even when fused to poorly folding peptides, was reported in 2006[12] Many other mutations have been made, including color mutants; in particular, blue fluorescent protein (EBFP, EBFP2, Azurite, mKalama1), cyan fluorescent protein (ECFP, Cerulean, CyPet), and yellow fluorescent protein derivatives (YFP, Citrine, Venus, YPet). BFP derivatives (except mKalama1) contain the Y66H substitution. The critical mutation in cyan derivatives is the Y66W substitution, which causes the chromophore to form with an indole rather than phenol component. Several additional compensatory mutations in the surrounding barrel are required to restore brightness to this modified chromophore due to the increased bulk of the indole group. The red-shifted wavelength of the YFP derivatives is accomplished by the T203Y mutation and is due to π-electron stacking interactions between the substituted tyrosine residue and the chromophore. These two classes of spectral variants are often employed for fluorescence resonance energy transfer (FRET) experiments. Genetically-encoded FRET reporters sensitive to cell signaling molecules, such as calcium or glutamate, protein phosphorylation state, protein complementation, receptor dimerization, and other processes provide highly specific optical readouts of cell activity in real time.
Semirational mutagenesis of a number of residues led to pH-sensitive mutants known as pHluorins, and later super-ecliptic pHluorins[13]. By exploiting the rapid change in pH upon synaptic vesicle fusion, pHluorins tagged to synaptobrevin have been used to visualize synaptic activity in neurons
Redox sensitive versions of GFP (roGFP) were engineered by introduction of cysteines into the beta barrel structure[14]. The redox state of the cysteines determines the fluorescent properties of roGFP The nomenclature of modified GFPs is often confusing due to overlapping mapping of several GFP versions onto a single name. For example, mGFP often refers to a GFP with an N-terminal palmitoylation that causes the GFP to bind to cell membranes. However, the same term is also used to refer to monomeric GFP, which is often achieved by the dimer interface breaking A206K mutation.[15] Wild-type GFP has a weak dimerization tendency at concentrations above 5 mg/mL. mGFP also stands for "modified GFP," which has been optimized through amino acid exchange for stable expression in plant cells.
STRUCTURE
|
GFP has a typical beta barrel consisting of with Inward-facing sidechains of the barrel induce specific cyclization reactions in the that lead to chromophore formation. This process of post-translational modification is referred to as maturation. The hydrogen-bonding network and electron-stacking interactions with these sidechains influence the color of wtGFP and its numerous derivatives. excludes solvent molecules, protecting the chromophore fluorescence from quenching by water.