Hen Egg-White (HEW) Lysozyme
From Proteopedia
Contents |
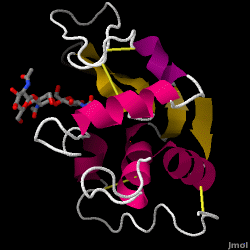
Lysozyme - also known as muramidase - is a powerful enzyme found in abundance in tears, saliva, and human milk. In humans, it is encoded in the LYZ gene. Since it is a small, easily available, and highly stable protein, it has been subject to extensive research regarding its function and structure.
- Tail-associated lysozyme is expressed in bacteriophage T4 plays a role in digesting the peptidoglycan layering in lysis from within[1].
Introduction
Lysozyme acts as a non-specific defense against bacteria and fungi. It is a component of the innate immune system, and is an important part of an infant's diet to ward off diarrhea. It is an enzyme known for its ability to degrade the polysaccharide architecture of many kinds of cell walls, normally for the purpose of protection against bacterial infection[2]. The structure of hen egg white (HEW) lysozyme, the focus of this article, is shown on the right. The antibacterial activity of hen egg white was first described by Laschtschenko in 1909[3]. It was characterized and named “lysozyme” by Alexander Fleming, the same person credited for the discovery of penicillin[4]. Discovery of the enzymatic activity was by accident; during the unrelated experiment, nasal drippings were inadvertently introduced to a petri dish containing a bacterial culture, which culture consequently exhibited the results of an as yet unknown enzymatic reaction. The observation of this unknown reaction led to further research on the components of this reaction as well as to the corresponding identification of the newfound "lysozyme." In 1965, David C. Phillips and coworkers determined the three-dimensional structure of lysozyme at 2 Å resolution [5]. Phillips' work was especially groundbreaking since Phillips had managed to successfully elucidate the structure of an enzyme via X-ray crystallography - a feat that had never before been accomplished[6][7]. Phillips' research also led to a structure-based hypothesis of its mechanism of action
[8].
Function
The primary function of lysozyme is to hydrolyze the bonds between the sugar molecules in the peptidoglycan layer of bacterial cell walls. Peptidoglycan is a rigid structure that provides support and protection to bacterial cells. By cleaving these bonds, lysozyme weakens the bacterial cell wall, leading to the rupture and death of the bacterium.
Lysozyme is particularly effective against Gram-positive bacteria, which have a relatively thick peptidoglycan layer. Gram-negative bacteria, on the other hand, have an outer membrane that provides additional protection, making them less susceptible to lysozyme.
In addition to its antimicrobial activity, lysozyme also plays a role in other physiological processes. It contributes to the maintenance of healthy epithelial tissues, such as those lining the respiratory and gastrointestinal tracts. Lysozyme helps prevent bacterial overgrowth and infection in these areas by inhibiting the growth of certain bacteria.
Lysozyme is widely distributed in nature and can be found in various organisms, including humans, animals, and plants. It is particularly abundant in the secretions of the lacrimal glands (tears) and salivary glands, where it helps protect the eyes and oral cavity from bacterial infections.
Due to its antimicrobial properties, lysozyme has been used in various applications, such as a natural food preservative and an additive in certain personal care products. It has also been studied for its potential therapeutic applications, including in the development of antimicrobial agents and the treatment of certain infections.
Chemical activity
The particular substrate of preference for this cleavage type is a (NAG-NAM)₃ hexasaccharide, within which substrate occurs the cleaving target glycosidic bond, NAM₄-β-O-NAG₅. The individual hexasaccharide binding units are designated A-F, with NAM₄-β-O-NAG₅ glycosidic bond cleavage preference corresponding to a D-E unit glycosidic bond cl
Lysozyme is known for damaging bacterial cell walls by catalyzing the hydrolysis of 1,4-beta-linkages between N-acetylmuramic acid (NAM) and N-acetyl-D-glucosamine (NAG) residues in peptidoglycan, and between N-acetyl-D-glucosamine residues in chitodextrins. In this way, lysozyme is efficient in lysing the cell walls of both bacteria and fungi. The location of cleavage for lysozyme on this architectural theme is the β(1-4) glycosidic linkage connecting the C1 carbon of NAM to the C4 carbon of NAG.
The particular substrate of preference for this cleavage type is a (NAG-NAM)₃ hexasaccharide, within which substrate occurs the cleaving target glycosidic bond, NAM₄-β-O-NAG₅. The individual hexasaccharide binding units are designated A-F, with NAM₄-β-O-NAG₅ glycosidic bond cleavage preference corresponding to a D-E unit glycosidic bond cleavage preference. Depending on the organism from which lysozyme is obtained, hydrolysis of the glycosidic bond proceeds with retention of configuration at the anomeric carbon (hen egg white) or with inversion (goose, phage T4).
Lysozyme efficiently acts on long (NAG-NAM) or (NAG) polymers. As the chain length gets smaller than six monomers, the catalytic rates drop substantially; in fact, trisaccharides act as competitive inhibitors by binding to the active site in a non-productive register.
|
Mechanism
Hydrolysis of glycosidic bonds by hen egg white lysozyme proceeds with retention of configuration. In 1953, Koshland [17] suggested that in general, retention of configuration implies a double-displacement mechanism (while inversion of configuration implies single displacement). For decades, two competing mechanistic hypotheses (Phillips[8]: dissociative mechanism with oxocarbenium intermediate; Koshland: two-step associative mechanism with covalent enzyme complex as intermediate) were considered, with data from 2001[15] tipping the scale toward the existence of a covalent intermediate. The absence of a substrate complex structure certainly contributed to difficulties distinguishing between possible mechanisms, as did the existence of two distinct mechanisms (retention and inversion of configuration) within the same structural family of enzymes (e.g. hen vs goose enzyme).
Lysozyme hydrolyzes a glycoside (hence the familial classification of lysozyme as a glycosylase[18]), which corresponds to the conversion of an acetal to a hemiacetal. The reaction proceeds in two steps as shown in the figure above. In the first step, Asp 52 acts as nucleophile and part of the sugar is the leaving group. In the second step, water acts as nucleophile and Asp 52 acts as leaving group. Both steps invert the configuration at the anomeric carbon, leading to an overall retention of configuration. Glu 35 acts as an acid in the first step (protonating the sugar the the glycosidic bond to make it a better electrophile) and as a base in the second step (deprotonating water to make it a better nucleophile). While the figure shows some of the sugars in a boat conformation to emphasize the inversion of configuration, these are not observed experimentally but are rather found in a chair conformation.
Applications of Lysozyme
Since lysozyme has been widely recognized for its antibacterial and antifungal properties, it has a wide variety of uses both in biochemical and pharmaceutical applications. In molecular biology, lysozyme is often used in the alkaline-lysis procedure for extracting and isolating plasmid DNA. It is used extensively in the pharmaceutical field for destroying gram-positive bacteria, and can be used to support already-existing immune defenses to fight bacterial infections. This enzyme is particularly important for preventing bacterial diseases in infants. Because of its antibacterial properties, lysozyme can also be used in the food industry to help prevent spoilage of foods.
3D structures of lysozome
See Also
- Lysozyme
- Retaining Glycoside Hydrolases
- Molecular Playground/Lysozyme
- User:Judy Voet/Lysozyme
- Lysozyme (Arabic)
- Lysozyme (hebrew)
References
- ↑ Nakagawa H, Arisaka F, Ishii S. Isolation and characterization of the bacteriophage T4 tail-associated lysozyme. J Virol. 1985 May;54(2):460-6. PMID:3157805 doi:10.1128/JVI.54.2.460-466.1985
- ↑ Ragland SA, Criss AK. From bacterial killing to immune modulation: Recent insights into the functions of lysozyme. PLoS Pathog. 2017 Sep 21;13(9):e1006512. doi: 10.1371/journal.ppat.1006512., eCollection 2017 Sep. PMID:28934357 doi:http://dx.doi.org/10.1371/journal.ppat.1006512
- ↑ Laschtschenko,P. (1909) Über die keimtötende und entwicklungshemmende Wirkung von Hühnereiweiss. Z. Hyg. Infektionskrankh.,64,419-427.
- ↑ Fleming, A. (1922) On a remarkable bacteriolytic element found in tissues and secretions. Proc.Roy.Soc.(London),93,306-317.
- ↑ Blake CC, Koenig DF, Mair GA, North AC, Phillips DC, Sarma VR. Structure of hen egg-white lysozyme. A three-dimensional Fourier synthesis at 2 Angstrom resolution. Nature. 1965 May 22;206(986):757-61. PMID:5891407
- ↑ Bugg, T. 1997. An Introduction to Enzyme and Coenzyme Chemistry. Blackwell Science Ltd., Oxford
- ↑ Earliest Solutions for Macromolecular Crystal Structures.
- ↑ 8.0 8.1 Blake CC, Johnson LN, Mair GA, North AC, Phillips DC, Sarma VR. Crystallographic studies of the activity of hen egg-white lysozyme. Proc R Soc Lond B Biol Sci. 1967 Apr 18;167(1009):378-88. doi:, 10.1098/rspb.1967.0035. PMID:4382801 doi:http://dx.doi.org/10.1098/rspb.1967.0035
- ↑ Image from: http://www.vuw.ac.nz/staff/paul_teesdale-spittle/essentials/chapter-6/proteins/lysozyme.htm
- ↑ Richardson JS. Early ribbon drawings of proteins. Nat Struct Biol. 2000 Aug;7(8):624-5. doi: 10.1038/77912. PMID:10932243 doi:http://dx.doi.org/10.1038/77912
- ↑ http://mcdb-webarchive.mcdb.ucsb.edu/sears/biochemistry/tw-enz/lysozyme/HEWL/lysozyme-overview.htm
- ↑ Blake CC, Koenig DF, Mair GA, North AC, Phillips DC, Sarma VR. Structure of hen egg-white lysozyme. A three-dimensional Fourier synthesis at 2 Angstrom resolution. Nature. 1965 May 22;206(986):757-61. PMID:5891407
- ↑ Johnson LN, Phillips DC. Structure of some crystalline lysozyme-inhibitor complexes determined by X-ray analysis at 6 Angstrom resolution. Nature. 1965 May 22;206(986):761-3. PMID:5840126
- ↑ Phillips (1966) Scientific American 215, 76-90
- ↑ 15.0 15.1 Vocadlo DJ, Davies GJ, Laine R, Withers SG. Catalysis by hen egg-white lysozyme proceeds via a covalent intermediate. Nature. 2001 Aug 23;412(6849):835-8. PMID:11518970 doi:10.1038/35090602
- ↑ Davies, Withers and Vocadlo (2009) The Chitopentaose Complex of a Mutant Hen Egg-White Lysozyme Displays No Distortion of the –1 Sugar Away from a 4C1 Chair Conformation, Australian Journal of Chemistry 62(6) 528-532
- ↑ Koshland, D. E. (1953). Biol. Rev. 28, 416–436
- ↑ https://bio.libretexts.org/Bookshelves/Biochemistry/Book%3A_Biochemistry_Online_(Jakubowski)/07%3A_CATALYSIS/B._Mechanisms_of_Enzyme-Catalyzed_Reactions/B2.__Lysozyme
Proteopedia Page Contributors and Editors (what is this?)
Karsten Theis, Michal Harel, Anne Goodling, Alexander Berchansky, Eric Martz, Joel L. Sussman, Karl Oberholser, Jaime Prilusky, John Ripollone, Daniel Kreider, Judy Voet, John S. de Banzie