Electron density maps
From Proteopedia
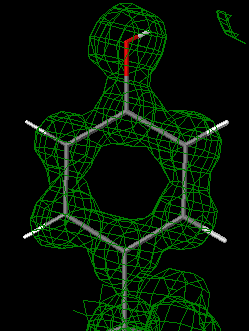
88% of the macromolecular structures available from the Protein Data Bank (PDB) were determined by X-ray crystallography (as of July, 2021). The direct results of crystallographic experiments are electron density maps[1]. The atomic model is the authors' interpretation of the map[1]. Examining the correspondence between the electron density map and the published molecular model reveals regions of uncertainty in the model.
Contents |
Crystallography Produces Electron Density Maps
An X-ray crystallographic experiment produces an electron density map for the average unit cell of the protein crystal[1]. The amino acid (or nucleotide) sequence of the crystallized polymer(s) is known in advance. The crystallographer fits the atoms of the known molecules into the electron density map, and refines the model and map to the limits of the resolution of the crystal (which is limited by the level of order or disorder in the crystal). The crystallographer then deposits a model of the asymmetric unit of the crystal in the PDB, along with the experimental diffraction data (intensities of the X-ray reflection spots, or "structure factor amplitudes"). From these combined, the electron density map can be reconstructed.
Why Look At Electron Density Maps?
Examining the correspondence between the published model PDB file and the electron density map (EDM) provides much clearer insight into the uncertainties in the model than does merely examining the model itself[2] (see also Quality assessment for molecular models). In addition to examining the map showing all features (2Fo-Fc), it is revealing to examine the difference map (Fo-Fc), which shows where the model fails to account for the map.
What are Electron Density Maps, and how can you obtain them?
Electron density values fill the 3-dimensional space of the map (see this interactive visualization). The term "map" is historical; the three-dimensional electron density was plotted on several pages, section by section, with contour lines corresponding to the value of the density at that location. These plots were called a map, in reference to topographical maps. For a paper showing several examples of maps printed in sections and overlayed, see a paper on tRNA structure.
The standard deviation of all density values, sigma, is used to describe the strength of features in a map. For example, a "3 sigma peak" is a feature that has a density value higher than three sigma over the average value, which typically is set to zero.
Electron density maps are available for most PDB files, e.g. from PDBe: at the page titled with the entry ID (4 characters), click on Downloads and look for "EDS map" and "EDS difference map". For some structures deposited before 2008, the diffraction data were not deposited and thus the electron density maps are not available. In 2008, the wwPDB began requiring that the data be deposited along with the model[3].
2Fo-Fc Map
An "all features" map, 2Fo-Fc, is the best way to calculate an estimate of the true electron density from diffraction data and atomic model. (It is called 2Fo-Fc because the calculation involves combining the observed diffraction data, Fo, with the diffraction data calculated from the atomic model, Fc, in a way that gives the least-biased result). Typically contoured at 1 sigma, it shows how well the observed density fits around the atomic model[2]. Ideally, a feature present in the crystal but not yet included in the model will show up with half the signal strength compared to features present in the crystal and included in the model. Likewise, spurious features included in the model will show up with half signal strength. This difference between real density and 2Fo-Fc density is called model bias, and is related to using the model (and the experimental data) to judge the correctness of the model, a bit of a circular argument.
- The example at right shows the first report, in 2021, of a new kind of covalent protein crosslink, a lysine-cysteine NOS bond between the sidechains of the two amino acids in 6zx4[4]. Crystal structures reported before the recognition of this type of crosslink will have overlooked the oxygen atom, as appears likely the case for 3u7z published in 2011. In contrast, the map shows clearly the absence of an oxygen between the nitrogen and sulfur in 6vb2 (similar N-S distances of 2.6-2.7 Å and similar resolutions of 1.3-1.4 Å).
2Fo-Fc maps are most useful in an intermediate stage of model building, when the model is already quite good but still missing major features (such as well-defined side-chains). There are more sophisticated tools (called omit maps[2]) in the final stages of refinement to complete the model in less obvious areas. At the end of the refinement, the 2Fo-Fc map should not reveal any glaring omissions but might show weakness in areas of the model that are less well defined (typical examples are the amino and carboxy termini, flexible loops, glycosylation sites, weakly bound ligands and water molecules). Likely errors are represented by (i) substantial density containing no atom, or (ii) atoms with little or no density.
Isomesh
The figures on this page include isomeshes. The isomesh is a mesh representing an isosurface. An isosurface is a surface placed at a specific value of a continuous parameter, so that it represents the boundary between higher and lower values of that parameter. Perhaps a topographic map is a more familiar example, where each isoline is a contour line joining positions with the same elevation. In an electron density map, an isomesh joins positions with the same electron density, for example, 1 sigma.
Disorder and atomic displacment parameters (temperature factors)
Different parts of a crystal structure can show different degrees of order. In a perfect crystal, every asymetric unit would look exactly the same, and not change atomic positions over time. In a real crystal, there is disorder. For example, side chains on the surface of the protein are often disordered (have multiple conformations), while side chains in the hydrophobic core are typically well-ordered (have a single conformation). It is also possible for one domain to be highly ordered (often the biggest domain with many contacts in the crystal) while another is less ordered (e.g. a domain loosely attached to the remainder of the structure).
A highly ordered atom will contribute a sharp peak to the electron density, while a less-ordered atom will contribute a broader and lower peak which is more difficult to distinguish from experimental noise. The atomic displacement parameters model this degree of order (with low values corresponding to ordered atoms and high values corresponding to disordered atoms). Thus by coloring the atomic model by disorder (also termed temperature factor or B factor), you can see which parts of a structure are most highly ordered without actually displaying the electron density. You can also see which parts of the atomic model have high certainty (those in highly-ordered regions with clear electron density) and which were difficult to model appropriately (those in less-ordered regions with features barely rising above the noise). In FirstGlance, in the Tools tab, "Local Uncertainty" colors by temperature factor.
Fo-Fc Difference Map
Fo-Fc is a "difference map". It shows where the experimental density and the atomic model disagree[2]. If the atomic model fitted the experimental density perfectly (and there was no experimental noise), the difference map would have no densities. Typically, it has negative densities (atoms in the model where there is no electron density, isomesh conventionally colored red) and positive densities (electron density where there is no atom in the model, isomesh typically colored blue or green). The isomesh typically represents the boundaries at ±3 sigma, namely, regions with a substantial level of disagreement. Because ±3 sigma is a relative measure, there will always be features in the Fo-Fc Difference Map. When the atomic model contains glaring mistakes, these peaks will correspond to real features. When the atomic model agrees optimally with the electron density, these peaks will mostly correspond to experimental noise.
Visualizing Electron Density Maps
Crystallographers generally use "heavy duty" visualization and modeling software such as Coot or PyMOL, which require considerable practice to use effectively, and offer a great deal of flexibility for model building.
A much easier way to view electron density maps is to use FirstGlance in Jmol. After you load your PDB ID, go to the Tools tab and click Density Maps for instructions. You can view the interactive 3D map for any residues that you specify, in a single click, without any command language. Any view in FirstGlance, including electron density maps, can be saved as a static image (see examples above on this page), or as an animation ready to drop into Powerpoint. To see examples of such animations in slides, check out tinyurl.com/movingmolecules.
Density map viewers are also available at each of the 3 branches of the wwPDB. They are somewhat more complex and technical than the map viewer in FirstGlance. These viewers are also capable of saving animations, although the interfaces are more technical than what FirstGlance offers.
Examples
In Proteopedia
- User:Karsten Theis/Electron density explains and illustrates how to display the electron density map in Jmol for any selected portion of a crystallographic structure, at various sigma levels.
- Some of the green links in Garman lab: Interconversion of lysosomal enzyme specificities show electron density maps for ligands.
Outside of Proteopedia
- Electron Density: Cloud vs. Isomesh "Map" shows a "raw" electron density map with buttons to hide densities below various sigma "noise" levels. It also shows the isomesh at 1.0 sigma, and the atomic model fitted to the isomesh.
- Electron Density Maps shows the map for bacterial flagellin, resolution 2.0 Å, and shows how the temperature/B-factor relates to the map in areas of low and high temperature.
See Also
Within Proteopedia
- Hydrogen in macromolecular models shows an example of hydrogens visible in an electron density map at 0.69 Å resolution.
- X-ray crystallography
- Resolution
- Electron cryomicroscopy which includes an overview of the resulting Coulomb potential maps.
Outside of Proteopedia
Acknowledgement
Thanks to Amr A. Alhossary for identifying examples of putative Lys-Cys NOS crosslink bonds.
References
- ↑ 1.0 1.1 1.2 Wlodawer A, Minor W, Dauter Z, Jaskolski M. Protein crystallography for non-crystallographers, or how to get the best (but not more) from published macromolecular structures. FEBS J. 2008 Jan;275(1):1-21. doi: 10.1111/j.1742-4658.2007.06178.x. Epub 2007, Nov 23. PMID:18034855 doi:http://dx.doi.org/10.1111/j.1742-4658.2007.06178.x
- ↑ 2.0 2.1 2.2 2.3 Lamb AL, Kappock TJ, Silvaggi NR. You are lost without a map: Navigating the sea of protein structures. Biochim Biophys Acta. 2015 Apr;1854(4):258-68. doi: 10.1016/j.bbapap.2014.12.021., Epub 2014 Dec 29. PMID:25554228 doi:http://dx.doi.org/10.1016/j.bbapap.2014.12.021
- ↑ Berman HM. Synergies between the Protein Data Bank and the community. Nat Struct Mol Biol. 2021 May;28(5):400-401. doi: 10.1038/s41594-021-00586-6. PMID:33963295 doi:http://dx.doi.org/10.1038/s41594-021-00586-6
- ↑ Wensien M, von Pappenheim FR, Funk LM, Kloskowski P, Curth U, Diederichsen U, Uranga J, Ye J, Fang P, Pan KT, Urlaub H, Mata RA, Sautner V, Tittmann K. A lysine-cysteine redox switch with an NOS bridge regulates enzyme function. Nature. 2021 May 5. pii: 10.1038/s41586-021-03513-3. doi:, 10.1038/s41586-021-03513-3. PMID:33953398 doi:http://dx.doi.org/10.1038/s41586-021-03513-3