Ribbon drawing of the "TIM barrel" fold
Introduction
Triose Phosphate Isomerase (TPI or TIM) is a ubiquitous dimeric enzyme with a molecular weight of ~54 kD (27 kD per subunit) which catalyzes the reversible interconversion of the triose phosphate isomers and , an essential process in the glycolytic pathway. More simply, the enzyme catalyzes the , also referred to as PGAL. In regards to the two isomers, at equilibrium, roughly 96% of the triose phosphate is in the DHAP isomer form; however, the isomerization reaction proceeds due to the rapid removal of GAP from the subsequent reactions of glycolysis. The TPI tertiary structure is the classic example of the "TIM barrel" fold (see image at left). The TPI structure is shown on the right (PDB entry 2ypi) in complex with the inhibitor 2-phosphoglycolic acid (PGA), which is bound to each of its two active sites. TPI is an example of a catalytically perfect enzyme, indicating that for almost every enzyme-substrate encounter, a product is formed and that this interaction is limited only by the substrate diffusion rate. See Glycolysis Enzymes and Isomerases.
In addition to its role in glycolysis, TPI is also involved in several additional metabolic biological processes including gluconeogenesis, the pentose phosphate shunt, and fatty acid biosynthesis. A point mutation to a glutamate residue (Glu104) of TPI results in triose phosphate isomerase deficiency, an autosomal recessive inherited disorder characterized by an increased accumulation of DHAP in erythrocytes. Structurally, this point mutation abolishes TPI’s ability to dimerize, subsequently inhibiting its catalytic activity. More details in
Mechanism
TPI catalyzes the transfer of a hydrogen atom from carbon 1 to carbon 2, an intramolecular oxidation-reduction reaction. This isomerization of a ketose to an aldose proceeds through an cis-enediol(ate) intermediate. This isomerization proceeds without any cofactors and the enzyme confers a 109 rate enhancement relative to the nonenzymatic reaction involving a chemical base (acetate ion)[1].
Isomerization reaction catalyzed by TPI
|
Kinetic constants of Triose Phosphate Isomerase
|
Acid-Base Catalysis
The mechanism of TPI has been extensively studied by prominent enzymologists for several decades leading to several different proposed mechanisms of catalysis. The original "Classic" mechanism put forth by Knowles and co-workers is outlined in the mechanism provided below.[2]
Classic Mechanism proposed by Knowles and co-workers
TPI carries out the isomerization reaction through an acid-base-mediated mechanism involving , each of which . First, the DHAP or GAP substrate is initially attracted to the enzyme active site through electrostatic interactions between the negatively charged phosphate group of the substrate and the positively charged Lys12, with the resulting interaction stabilizing the substrate.
According to the "classic" mechanism, Glu165 plays the role of the general base catalyst by abstracting a proton from the pro(R) position of carbon 1 of DHAP or the C-2 proton of GAP. However, the carboxylate group of Glutamate 165 alone does not possess the basicity to abstract a proton and requires
His95, the general acid, to donate a proton to stabilize the negative charge building up on C-2 carbonyl oxygen, effectively stabilizing the planar endediol(ate) intermediate. Lys12 and Asn11 also function to stabilize the negative charge which builds up on this intermediate.
At this point in the mechanism, Glutamate 165 acts as a general acid by donating its proton to the neighboring C-2, while Histidine 95 now acts as a general base by abstracting a proton from the hydroxyl group of C-1. The final step in the reaction is the formation of the GAP isomer product while glutamate and histidine are returned to their original forms, regenerating the enzyme. In studies using tritium labeled DHAP, Knowles observed only ~ 6% intramolecular transfer of the 3H label to the GAP product. In explaining this result, Knowles argued that the hydrogen bound to the Glu165 was in equilibrium with those in bulk solvent. Additionally, the reaction mechanism of the methylglyoxal forming enzyme methylglyoxal synthase (MGS) is believed to be similar to that of triosephosphate isomerase. Both enzymes utilize DHAP to form an enediol(ate) phosphate intermediate as the first step of their reaction pathways; however, the second catalytic step in the MGS reaction pathway features the elimination of phosphate and collapse of the enediol(ate) to form methylglyoxal rather then reprotonation to form the GAP isomer as seen in TPI.[3]
The Enediol(ate) Intermediate as a Kinetic Barrier
The formation and stabilization of the enediol(ate) intermediate has been a source of great debate amongst enzymologists. This is due to the fact that the formation of the enediol(ate) intermediate presents a large thermodynamic barrier involving the abstraction of the α-proton from the carbon acid substrate, an unfavorable process due to the lack of acidity and high pKa of the C1 proton of the substrate. The "classic" mechanism (shown above) put forth by Knowles and co-workers stated that the kinetic barrier is overcome by the concerted deprotonation of the C1 and the protonation of the C2 carbonyl oxygen which would effectively allow for equivalent free energies for each species shown, thus promoting an equilibrium constant near unity.[4] This concept of preferential binding arising from matching of the reaction energy barriers is a common theme of enzyme catalysis [5].
Free-Energy Profile for the Reaction Catalyzed by Triose phosphate Isomerase
Low-Barrier Hydrogen Bond in the TPI Mechanism
An additional explanation of the TPI mechanism proposed by Cleeland and Kreevoy stipulates the formation of a Low-barrier hydrogen bond[6]. Support for this LBHB arose from the rare observation of a hydrogen bond between the carbonyl oxygen of the substrate and a neutral histidine. It was reasoned that a neutral histidine was required to match the pKa of the enediol, a requirement for the formation of a shorter and stronger LBHB (pKa's ~ 14). It was rationalized that this strengthened hydrogen bond and ideal geometry would effectively speed up the enolization reaction. Structural evidence for this LBHB was found in a 1.2 Å crystal structure of TIM complexed with DHAP demonstrating an extremely short hydrogen bond (2.6 Å) between His95 and O2 of DHAP [7]. Under the mechanism stipulating a LBHB between His95 and O2 of DHAP, Glu165 would catalyze all proton transfers between C1 and C2, while His95 would act as an electrophilic catalyst by forming a close, stabilizing LBHB with the cis-enediolate intermediate.
Proposed LBHB between Histidine and Enediol Intermediate.
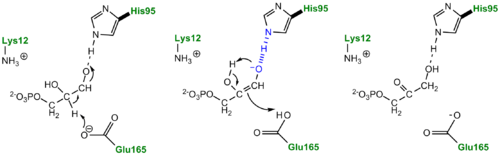
More recently a series of NMR experiments carried out by Mildvan and co-workers have shed light onto an alternative "Criss-cross" mechanism involving a LBHB between the catalytic Glu165 and the O1 oxygen of the substrate. This mechanism stipulates the His95 side chain does not directly transfer protons, this rather being accomplished entirely by Glu165. Support for this mechanism was provided by Richard and coworkers who carried out tritium labeling experiments demonstrating a significant amount of intramolecular transfer (49%) of the 3H label from substrate (DHAP) to product (GAP)[8]. Using phosphoglycolohydroxamate (PGH), a mimic of the enediol(ate) intermediate, a 14.9 ppm chemical shift (6 ppm downfield) as well as a deuterium fractionation factor of 0.38 was observed with the TIM-PGH complex, corresponding to a highly deshielded proton involved in a LBHB between Glu165 and the hydroxamate oxygen of PGH. Conversely, the same NMR study found an additional hydrogen bond between the N-ε proton of His95 and the carbonyl oxygen of PGH; however, its chemical shift of 13.5 (0.4 ppm downfield from free enzyme) and fractionation factor of 0.71 indicated this was a strong H-bond, but not a LBHB.[9].
LBHB between Glu165 and DHAP The formation of the LBHB between Glu165 and O1 of the inhibitor PGH is due to the matching of p
Kas and the alternative mechanism suggests that Glu-165, in addition to its role in initially abstracting the proton from the substrate, may also shuttle protons to and from the oxygens in the intermediate. Also, the "criss-cross" mechanism implies that, by donating a normal hydrogen bond, the role of His95 is to polarize the carbonyl oxygen and lower the p
Ka of PGH in order to facilitate subsequent proton abstraction
[10]. It has been argued that that the LBHB formed between Glu165 and PGH is a consequence of using the inhibitor PGH, whose hydroxamate p
Ka of 9 better matches Glu165 then His95, and that the biological reaction would instead see the enediol forming a LHBH with His95, as mentioned above. Overall, the mechanism employed by TPI has yet to be completely solved and recent NMR studies involving both WT and mutant TPI enzymes have revealed contributions from both the "classic" and "criss-cross" mechanisms.
Alternative "Criss-Cross" TPI Mechanism Involving LBHB Between Glu165 and O1 of the Intermediate
Inhibitors of Triose Phosphate Isomerase
Inhibitors of Triose Phosphate Isomerase
Although a well characterized enzyme, there are relatively few effective inhibitors of TPI. From a pharmaceutical perspective, if TPI structures differ greatly between humans and microorganisms such as Plasmodium or Trypanosoma, whose growth rely heavily or entirely on glycolysis, inhibition may be an attractive therapeutic target.[11] Two irreversible inhibitors, halo-acetone phosphate and glycidol phosphate (1,2-epoxypropanol-3-P), act by labeling active site residues, effectively abolishing enzymatic activity. Early biochemical studies involving glycidol phosphate have revealed the labeled residue to be the active site glutamate. There are several weak reversible inhibitors of TPI including 3-phosphoglycerate, glycerol phosphate and phosphoenol pyruvate, with Ki values ranging from 0.2-1.3 mM.[12] Additionally, several transition state analogues have been used to study the mechanism of TPI, including phosphoglycolohydroxamate(PGH)(Ki = 6-14 μM) and the phosphoglycolic acid (PGA)(Ki = 3 μM) and 2(N-formyl-N-hydroxy)aminoethyl phosphonate (IPP) [13]. PGA (also called 2PG) is believed to bind TPI as a trianion, undergoing tight active site binding through electrostatic interactions with both the neutral His95 and protonated Glu165 side chains. PGH (binding in the cis conformation) and IPP function by mimicking structural features of the natural DHAP and GAP substrates, respectively[14]. Specifically, PGH effectively mimics the planar enediol(ate)intermediate.
Structure & Function
Triose Phosphate Isomerase is a member of the all alpha and beta (α/β) class of proteins and it is a homodimer consisting of two sequence-identical subunits (chains) each comprising 247 amino acids. Each TPI monomer (chain) contains the full set of catalytic residues; however, the enzyme is only active in the oligomeric form.[15] Therefore, dimerization is essential for full function of the enzyme even though it is not believed that any cooperativity exists between the two active sites.[16] Each subunit contains 8 exterior alpha helices surrounding 8 interior beta strands, which form a (αβ) or more specifically a TIM barrel. The TIM barrel was originally named after TPI and is estimated to be present in 10% of all enzymes. Characteristic of most all TIM barrel domains is the presence of the enzyme's active site in the lower loop regions created by the eight loops that connect the C-termini of the beta strands with the N-termini of the alpha helices. TIM barrel proteins also share a structurally conserved phosphate binding motif, with the phosphate group found in the substrate or cofactors. [17].
In each chain, nonpolar amino acids pointing inward from the beta strands contribute to the hydrophobic core of the structure (). The alpha helices are amphipathic: their outer (water-contacting) surfaces are polar, while their inner surfaces are largely hydrophobic (). The loops are a mixture ().
Ω Loop 6
As mentioned earlier, TPI is considered a catalytically perfect enzyme and accomplishes this largely due to its ability to suppress or prevent undesired side reactions such as the decomposition of the enediol intermediate into methyl glyoxal and orthophosphate, a process which is 100 fold faster in solution than the desired isomerization. TPI is able to prevent this undesired reaction by trapping and stabilizing the charged endiol(ate) intermediate in the active site through the use of a flexible 11 residue Ω loop (omega loop) referred to as . Loop 6 spans residues 168-178[18], (numbering varies with species) and can be further divided into a 3-residue N-terminal hinge, a rigid hydrophobic lid spanning 5-residues and a 3-residue C-terminal hinge (.) The complete closure of this loop, a movement of roughly 7 Å for the tip of the loop (Cα of Thr172) and occurring on a microsecond timescale, is facilitated by several hydrogen bonding interactions between loop 6 and loop 7. These include H-bonds between the hydroxyl group of tyrosine 208 (loop 7) and the amine nitrogen of alanine 176 as well as between serine 211 (loop 7) and glycine 173. As mentioned above, the loop shuts when the enediol is present, effectively shielding both ligand and catalytic residues from solvent exposure, and reopens when the isomerization is complete.
Site-directed mutagenesis experiments substituting a Phenylalanine for the Tyrosine resulted in a 2400-fold decrease in catalytic activity[19]. It is believed the opening/closing of loop 6 and loop 7 is partially rate-limiting. Additionally, extensive mechanistic and kinetic experiments involving Trypanosoma brucei, a parasitic protist causing sleeping sickness in humans, has revealed the structural and functional importance of a proline residue at position 168 in conjunction with transmitting the signal of ligand binding to the conformational change of the catalytic glutamate residue (Glu167 in T.brucei) and the subsequent proper loop 6 closure.[20] Specifically, the proline residue is positioned at the beginning of loop 6 to aid in the catalytic glutamate side chain flipping from the inactive swung-out to the active swung-in conformation, facilitating the closure of the loop. Structurally, in the unliganded (open) conformation, the Glu-Pro peptide bond is in the energetically favored trans conformation; however, in the liganded (closed) conformation, the pyrrolidine ring of proline adopts a rare strained planar conformation (9 kJ/mol in vacuo), suggesting that the strain could be important for loop opening and product release upon completion of the reaction cycle.[21]
Entropic Effects of Ω Loop 6 Hinges
Similar to the loop spanning residues, the Ω loop 6 hinge residues share high sequence homology amongst species. The role of both the N- and C-terminal hinge regions of Ω loop 6 have been extensively studied including the replacement of conserved hinge residues with glycine, which resulted in a 2500-fold drop in kcat. The insertion of glycine into the hinge region significantly increases the flexibility of the loop due to glycine's conformational freedom, which in turn allows the loop to sample many more conformations. This has thermodynamic ramifications as these glycine-rich hinge mutants prompted a large entropy gain (+ΔS) compared to WT, effectively altering the entropic activation energy. Specifically, WT TPI is able to overcome the initial entropic gain (order to disorder), caused by dispelling water molecules from the active site, by forming a more ordered enzyme-substrate complex. Conversely, the glycine-rich hinge mutants again promote an initial entropy gain due to water loss but are unable to pay the entropic penalty due to their inability to bind substrate tightly. Since catalysis will only occur when the closed conformation has been sampled it reasons that the likelihood of sampling this conformation is greatly reduced with the glycine-rich hinge mutants, as evidence by a significant drop in kcat. As their overall functional role in the enzyme, the loop hinges act to limit the motion of the loop which effectively restricts the number of conformations accessible to the enzyme. In this manner, TPI acts like an entropy trap.
Why is the enzyme an obligate dimer?
An (residues 71-77) is found on each subunit. The loop extends from one subunit into a pocket near the active site of the other subunit. Notably, there is a hydrogen bond network involving Lys12 and Glu97 of one subunit and Thr75 of the other subunit. This may explain why the dimer is required for catalytic activity.
Disease
Triose Phosphate Isomerase Deficiency: Initially described in 1965, TPI deficiency is an autosomal recessive inherited disorder with characteristics ranging from chronic haemolytic anaemia, increased susceptibility to infections, severe neurological dysfunction, and often times death in early childhood.[22] The effects of TPI deficiency are most closely linked to a point mutation at the residue which results in the mutation. A common marker for TPI deficiency is the increased accumulation of DHAP in erythrocyte extracts as a result in the inability of the mutant enzyme to catalyze the isomerization to GAP. Recent evidence has indicated that the point mutation does not prove detrimental to the rate of catalysis of the enzyme, but rather effects the ability of the enzyme to dimerize.[23]
Role in Alzheimer's Disease: Recent discoveries in Alzheimer Disease research have indicated that amyloid beta-peptide induced nitro-oxidative damage promotes the nitrotyrosination of TPI in human neuroblastoma cells.[24] Nitrosylated TPI was found to be present in brain slides from double transgenic mice over-expressing human amyloid precursor protein as well as in Alzheimer's disease patients. Specifically, the nitrotyrosination occurs on , which are located in close proximity to the catalytic center, and this modification correlates with reduced isomerization activity. Additionally, Francesc Guix and colleagues have shown nitrosylated TPI contributed to the formation of large beta-sheet aggregates in vitro and in vivo.
Evolutionary Conservation
TPI has a roughly 50% sequence conservation from bacteria to humans.[25] The (2ypi) shows dramatic conservation around the catalytic site. Glu104 is also highly conserved, as are several residues in the interdigitating loop. Curiously, two Arg residues on the surface, distant from the dimer contact and the catalytic side, are also highly conserved. (See note about the conservation calculation[26].)
One specific example of sequence homology is that of loop 6 and loop 7 residues, whose structural contributions are discussed above. In a sequence alignment of 133 TIM sequences, two highly conserved motifs are noticed. First, 114 sequences in loop 6 contain the PXW sequence family (where X is I,L or V in 112 sequences or otherwise a T or K). Secondly, loop 7 contains a highly conserved YGGS motif; however, this motif is only found when the N-terminal hinge contains tryptophan.
3D Structures of triose phosphate isomerase
Triose phosphate isomerase 3D structures